- 1Frontiers Science Center for Deep Ocean Multispheres and Earth System, and Key Laboratory of Marine Chemistry Theory and Technology, Ocean University of China, Ministry of Education, Qingdao, China
- 2Laboratory for Marine Ecology and Environmental Science, Qingdao National Laboratory for Marine Science and Technology, Qingdao, China
- 3Marine Ecology, GEOMAR Helmholtz Centre for Ocean Research Kiel, Kiel, Germany
Ocean-related global change has strongly affected the competition between key marine phytoplankton groups, such as diatoms and dinoflagellates, especially with the deleterious consequency of the increasing occurrence of harmful algal blooms. The dominance of diatoms generally shifts toward that of dinoflagellates in response to increasing temperature and reduced nutrient availability; however, contradictory findings have also been observed in certain sea areas. A key challenge in ecology and biogeochemistry is to quantitatively determine the effects of multiple environmental factors on the diatom-dinoflagellate community and the related changes in elemental and biochemical composition. Here, we test the interplay between temperature, nutrient concentrations and their ratios on marine diatom-dinoflagellate competition and chemical composition using bi-algal competition experiments. The ubiquitous diatom Phaeodactylum tricornutum and dinoflagellate Prorocentrum minimum were cultivated semi-continuously, provided with different N and P concentrations (three different levels) and ratios (10:1, 24:1, and 63:1 molar ratios) under three temperatures (12, 18, and 24°C). The responses of diatom-dinoflagellate competition were analyzed by a Lotka-Volterra model and quantified by generalized linear mixed models (GLMMs) and generalized additive models (GAMs). The changes in nutrient concentrations significantly affected diatom-dinoflagellate competition, causing a competitive superiority of the diatoms at high nutrient concentrations, independent of temperature and N:P supply ratios. Interestingly, the effect amplitude of nutrient concentrations varied with different temperatures, showing a switch back toward a competitive superiority of the dinoflagellates at the highest temperature and at very high nutrient concentrations. The ratios of particulate organic nitrogen to phosphorus showed significant negative correlations with increasing diatoms/dinoflagellates ratios, while lipid biomarkers (fatty acids and sterols) correlated positively with increasing diatoms/dinoflagellates ratios over the entire ranges of temperature, N and P concentrations and N:P ratios. Our results indicate that the integration of phytoplankton community structure and chemical composition provides an important step forward to quantitatively understand and predict how phytoplankton community changes affect ecosystem functions and biogeochemical cycles in the ocean.
Introduction
The changes in hydrological conditions and climate warming have strongly influenced phytoplankton community composition, which consequently affects marine food webs and biogeochemical cycles as different phytoplankton groups have varying contributions to the ocean’s biological pump (Falkowski and Oliver, 2007). It has been increasingly realized that the pivotal roles of key phytoplankton groups in marine ecosystems relate with distinctive chemical composition of each group (Dalsgaard et al., 2003; Volkman, 2003; Weber and Deutsch, 2010; Shemi et al., 2016; Jo et al., 2021). C:N:P stoichiometry in marine phytoplankton primarily reflects the evolutionary inheritance, e.g., lower N:P molar ratios in diatoms compared to dinoflagellates (Quigg et al., 2003). Determining biologically and abiotically driven variability in phytoplankton C:N:P stoichiometry (particulate organic carbon to nitrogen (POC/PON) and particulate organic nitrogen to phosphorus (PON/POP) hereafter) is of critical importance for understanding ocean biogeochemical cycles and their interactions with environmental changes (Tyrrell, 1999; Martiny et al., 2013; Lee et al., 2021). Equally important is biochemical composition in phytoplankton. Certain phytoplankton-produced biomolecules (biomarkers), such as fatty acids (FAs) and sterols have been applied as trophic markers for tracing food web structures (Dalsgaard et al., 2003; Martin-Creuzburg and von Elert, 2009; Ruess and Müller-Navarra, 2019; Kohlbach et al., 2021) and as proxies for reconstructing phytoplankton community structure in the past and present-day ocean (Schubert et al., 1998; Zimmerman and Canuel, 2002; Wu et al., 2016; Wang et al., 2019). Ocean-related global change has significant effects on the chemical composition of phytoplankton (Guschina and Harwood, 2009; Hixson and Arts, 2016; Ding et al., 2019; Bi et al., 2020), e.g., showing up to a 83% increase in stoichiometric P:C in response to warming and a 76% increase in carbon-normalized contents of polyunsaturated fatty acids (PUFAs) in response to nutrient deficiency (Bi et al., 2017). Despite this increasing awareness of strong variations in the chemical composition of phytoplankton, little is known about the quantitative relationship between marine phytoplankton community structure and its chemical composition in the changing environments.
Diatoms and dinoflagellates are major phytoplankton groups in the ocean. Diatoms contribute as much as 40% of the total annual organic carbon production in the sea and occupy the base of the simple, ecologically efficient food web which is typical at high nutrient regimes with rapid changes of light conditions (Sommer et al., 2002; Armbrust et al., 2004). Adaptation of diatoms to such environments has resulted in their evolution of photosynthetic and metabolic machinery optimized to different nutrient and light regimes (Lavaud et al., 2004; Gao et al., 2018; Smith et al., 2019; Fisher et al., 2020). In contrast, dinoflagellates are favored following seasonal stratification and nutrient depletion in eutrophic coastal seas, fuel microbial food webs and have the potential to form harmful algal blooms (Margalef, 1978; Smayda, 2002; Sommer et al., 2002; Delwiche, 2007). Diatoms and dinoflagellates have distinct biochemical composition. For example, diatoms contain high levels of 16:1n-7, EPA (eicosapentaenoic acid, 20:5n-3) and brassicasterol/epi-brassicasterol, and high ratios of 16:1n-7/16:0 and EPA/DHA (docosahexaenoic acid, 22:6n-3), while characteristic FAs and sterols of dinoflagellates are 18:0; 18:1, DHA, and dinosterol (Dalsgaard et al., 2003; Volkman, 2003; Bi et al., 2014; Jiang et al., 2016). Such differences in biochemical composition have shown strong effects on trophic carbon transfer between phytoplankton and consumers (Müller-Navarra et al., 2000; Martin-Creuzburg and von Elert, 2009; Malzahn et al., 2010; Bi and Sommer, 2020). Also, on a long-term scale the shift in the diatom-dinoflagellate community have been reported in many areas of the ocean, which were driven by environmental changes induced by anthropogenic and climatic forcing (Schubert et al., 1998; Xing et al., 2016; Yuan et al., 2020).
Temperature and nutrients (N and P) are the most important factors controlling the diatom-dinoflagellate community (Glibert et al., 2011; Cross et al., 2015; Sunagawa et al., 2015; Cloern et al., 2016). The ocean is simulated to be warmed 5- to 7-fold from today to 2100 compared with the change from 1970 until today, according to the RCP8.5 scenario of the high emissions (Bindoff et al., 2019). The external supply of N and P to the ocean has increased, with a projected total input of 50:1 in N:P molar ratios to the ocean by 2050 (Moore et al., 2013). N:P ratios have strong variations, e.g., a molar ratio of 6:1 in the Southeast Pacific Gyre (Bonnet et al., 2008) and up to 100:1 in summer in certain areas of the East China Sea (ECS) (Wu et al., 2016). Warming, eutrophication and imbalanced N:P ratios have caused dramatic changes in the diatom-dinoflagellate community, which is typified by an increase in relative abundance of dinoflagellates compared to diatoms, with dinoflagellates becoming dominant together with diatoms or being the only dominant group (Xie et al., 2015; Liu et al., 2016; Siemering et al., 2016; Carreto et al., 2018).
However, contrasting observations have been obtained on the responses of diatom-dinoflagellate communities to temperature and nutrient availability changes. An increase in temperature generally enhances the abundance of dinoflagellates but not diatoms (Morse et al., 2013; Jiang et al., 2015; Xie et al., 2015); in contrast, an increase in the relative abundance of diatoms vs. dinoflagellates with increasing temperature has been found in different sea areas, such as the northeast Atlantic and North Sea (Hinder et al., 2012) and Chilean Patagonian fjord (Montero et al., 2017). While diatoms usually dominate at high nutrient conditions at both global and local scales (Aubry et al., 2004; Guo et al., 2014a; Rousseaux and Gregg, 2015; Mutshinda et al., 2016), some have reported the dominance of dinoflagellates at high nutrient conditions (Lotze and Milewski, 2004; Liu et al., 2016; Klais et al., 2017). An increasing N:P ratios often negatively correlates with the diatoms/dinoflagellates ratio (Zhang, 2009; Glibert et al., 2011; Guo et al., 2014b); however, a positive correlation has been also found in some coastal areas (Hodgkiss and Ho, 1997; Heisler et al., 2008; Xiao et al., 2013). Such seemingly contradictory findings on diatom-dinoflagellate competition can be largely attributed to the interplay between multiple environmental factors, especially between warming and eutrophication (Lewandowska et al., 2014; Cross et al., 2015; Xiao et al., 2018; Gerhard et al., 2019). While increasing attention has been devoted to experimentally study the effects of temperature and/or nutrient conditions on the diatom-dinoflagellate community (Wang et al., 2006; Lassen et al., 2010; Huang et al., 2012; Bach et al., 2020), our quantitative knowledge of these effects is still inadequate.
In this study, laboratory experiments were conducted to investigate the responses of a diatom-dinoflagellate community to the interactions between temperature, N and P concentrations and their ratios. In particular, we focus on quantitatively assessing the changes in elemental stoichiometry and lipid biomarkers associated with the shifts of community composition. We hypothesize that (1) dinoflagellates have the competitive superiority at high temperature, as well as at combined conditions of low temperature, high N:P supply ratios and a low nutrient regime; diatoms have the competitive superiority at combined conditions of low temperature, low N:P supply ratios and a low nutrient regime, as well as at low temperature and a high nutrient regime (Figure 1); and (2) the changes in elemental and biomarker composition can quantify the shifts in a diatom-dinoflagellate community under different temperature and nutrient conditions.
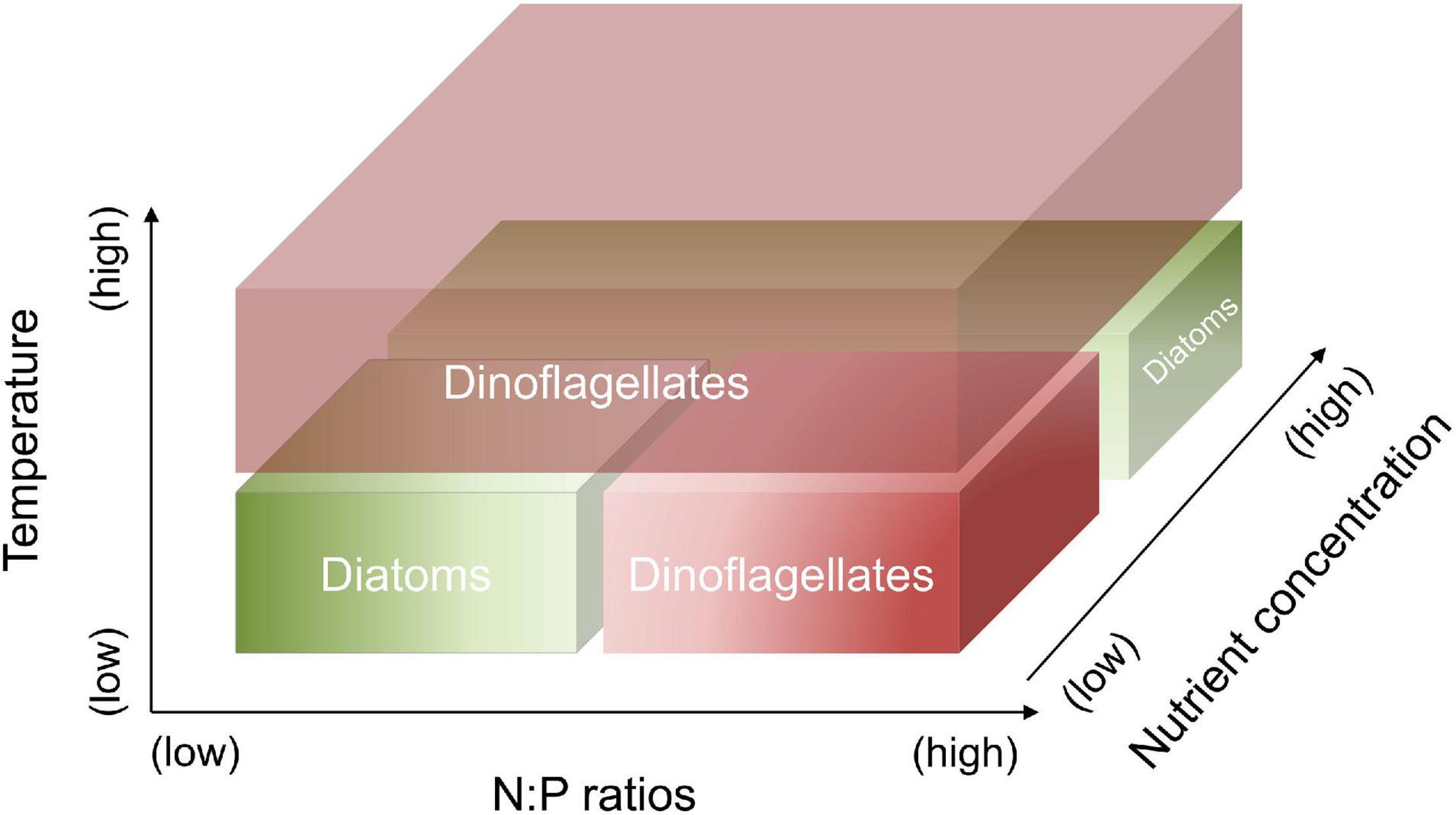
Figure 1. Hypothetic responses of diatoms vs. dinoflagellates to the changes in temperature, N:P supply ratios and nutrient concentrations. Dinoflagellates have the competitive superiority at high temperature, as well as at combined conditions of low temperature, high N:P supply ratios and a low nutrient regime. Diatoms have the competitive superiority at combined conditions of low temperature, low N:P supply ratios and a low nutrient regime, as well as at low temperature and a high nutrient regime.
Materials and Methods
Experimental Set-Up
The phytoplankton species used in the experiments were the diatom Phaeodactylum tricornutum (Code No.: MACC/B254, supplied by the Microalgae Culture Center at the Ocean University of China) and the dinoflagellate Prorocentrum minimum (Code No.: HYESL63, isolated from the ECS and supplied by Microalgae Germplasm Bank in the Second Institute of Oceanography, the Ministry of Natural Resources). Both monocultures and bicultures of the two species were exposed to a salinity of 37 psu and a light intensity of 100 μmol photons m–2 s–1 with a light:dark cycle of 12:12 h in temperature-controlled cabinets of 12, 18, and 24°C. The light intensity used in this study did not limit the growth of P. tricornutum and P. minimum (Beardall and Morris, 1976; Grzebyk and Berland, 1996). The chosen temperatures cover the optimal values for the growth of the two species (Grzebyk and Berland, 1996; Bojko et al., 2013), and the range of 6°C was set based on the ocean general circulation model under the scenarios of IPCC SRES A1F1 (Lewandowska et al., 2014). In bicultures, two species were mixed from the beginning on in the experiments. The culture medium was prepared according to the modified Provasoli’s medium (Provasoli, 1963; Ismar et al., 2008). Enrichment nutrient solutions were added to sterile filtered (0.2 μm pore size, Sartobran® P 300) sea water. Sodium nitrate and potassium dihydrogen phosphate were added to achieve the molar ratios of 10:1, 24:1, and 63:1 at three different nutrient concentration levels (Table 1). Sodium silicate was added to the medium at a concentration of 880 μmol L–1 in all treatments. At the normal nutrient level, N and P concentrations were comparable with those in the f/2 medium, which has been widely used as a general enriched seawater medium for culturing coastal marine algae (Harrison and Berges, 2005), while a 5-fold increase in N and P concentrations from the normal to high nutrient level covered the ranges of nutrient concentration changes in natural conditions, such as in the ECS (Xiao et al., 2018). The intermediate level of N:P supply ratios (24:1) was also consistent with that in the f/2 medium. The culture volume was 200 mL, and each treatment was replicated three times. All culture flasks were carefully agitated twice per day at a set time to minimize sedimentation. Prior to the experiments, the two species were maintained under the tested conditions for more than 12 generations to ensure that lipid composition of phytoplankton reflected acclimated responses (Burkhardt et al., 1999; Reinfelder, 2012).
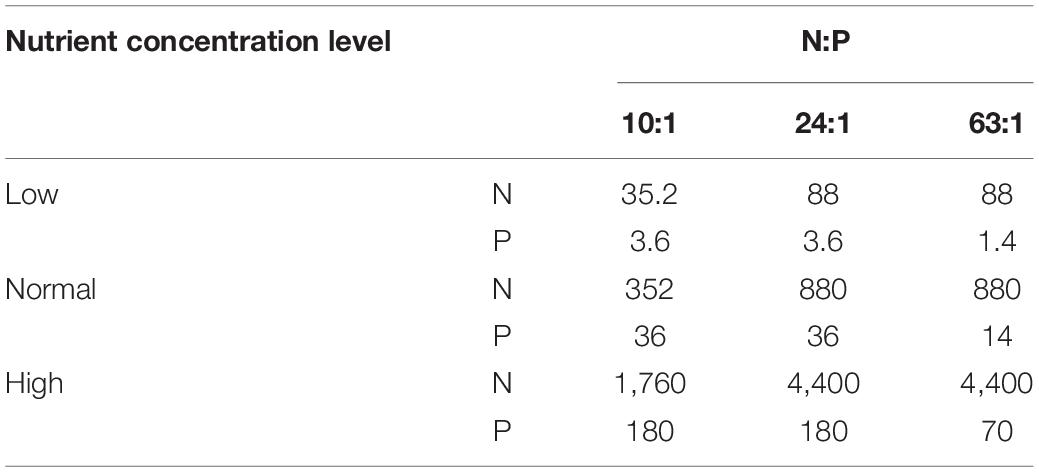
Table 1. N and P concentrations (μmol L–1) and their molar ratios in bi-algal competition experiments.
Batch cultures were first set for each treatment across a fully factorial combination of three temperatures (12, 18, and 24°C), three N:P supply ratios (molar ratios 10:1, 24:1, and 63:1) and three nutrient concentration levels. The observed maximal growth rate (μmax, d–1) was calculated based on cell numbers within the exponential growth phase of batch cultures (Supplementary Table 1). Once the early stationary phase was reached, semi-continuous cultures were initiated with the algae from batch cultures. For bicultures, the growth of the diatom P. tricornutum was used to determine the start of semi-continuous cultures. In semi-continuous cultures, the gross growth rate (μ, d–1) was set as 20% of μmax. The volume of the daily renewal medium was determined by multiplying the daily renewal rate (D, d–1; D = 1 – e–μ × t, where t is renewal interval which was 1 d in the experiments) by the culture volume (200 mL). As both species had different μmax in bicultures, μ of P. tricornutum was used to define the daily renewal rate. Steady state of semi-continuous cultures was assessed based on the net growth rate (r, d–1), i.e., the difference between μ and the loss rate (r = μ - D). In semi-continuous cultures, population losses result from the process of daily renewal incubation water, and the loss rate equals the renewal rate (D). At steady state in monocultures, μ was equivalent to D, and r was zero, while in bicultures, a zero net growth rate existed for both species if they coexisted, and for the dominant species when the other was driven to low abundances or excluded.
Sample Analysis
Algal cells were harvested at steady state in semi-continuous cultures. The following parameters were analyzed: Cell density, POC, PON, POP, FAs, and sterols. Cell density was measured daily during the experiments using an improved Neubauer hemacytometer (Glaswarenfabrik Karl Hecht GmbH) under a microscope (Olympus CX41) (Supplementary Figure 1). Algal cells for elemental and lipid biomarker analysis were harvested by filtration on pre-combusted and hydrochloric acid-treated GF/F filters (Whatman) at the same time. Filtration volumes were 15–30 mL depending on cell density. After filtration, all samples were frozen at -80°C until analysis.
The determination of POC and PON was carried out after Sharp (1974) by gas chromatography in an organic elemental analyzer (Thermo Flash 2000), with organic analytical standards atropine (Thermo Fisher Scientific) and a low organic content soil (Elemental Microanalysis Ltd.) as standards. POP was analyzed by converting organic phosphorus compounds to orthophosphate (Hansen and Koroleff, 1999) and measured colorimetrically at 880 nm with a spectrophotometer (HITACHI U-2910).
Lipid biomarkers (sterols and FAs) were measured according to Zhao et al. (2006). Briefly, freeze-dried samples were extracted with the mixture of dichloromethane and MeOH (3:1, vol/vol) for eight times, with C19 n-alkanol and nonadecanoic acid (19:0) as internal standards. After hydrolysis with 6% KOH in MeOH, the extracts were separated into polar and apolar fractions using silica gel chromatography. The polar lipid fraction (containing brassicasterol/epi-brassicasterol and dinosterol) was eluted with 22-mL dichloromethane/methanol (95:5, vol/vol) and dried under a gentle N2 stream. After elution, the polar fractions were silylated with 80 μL BSTFA (N, O-bis(trimethylsilyl)-trifluoroacetamide) at 70°C for 1 h. FAs in the acid fraction were derivatized to corresponding fatty acid methyl esters (FAMEs) with MeOH/HCl (95:5, 12 h at 70°C). Sterols and FAMEs were quantified based on internal standards on a gas chromatograph with flame ionization detection (GC-FID; Agilent Technologies 7890A). The GC columns for sterol and FA analysis were HP-1 (50 m × 0.32 mm i.d., 0.17-μm film thickness; Agilent J&W) and SP-2560 (100 m × 0.25 mm i.d., 0.20-μm film thickness; Supelco), respectively. Sterols were identified according lab standards, while FAs were identified with reference to commercially available standards, Supelco 37 component FAME mixture and Supelco Menhaden fish oil.
Data Analysis and Statistics
Species interactions were estimated by fitting Lotka-Volterra model (Eqs. 1 and 2):
where Ndiatom and Ndino (104 cells ml–1) represent cell density of the diatom P. tricornutum and the dinoflagellate P. minimum, respectively, during semi-continuous cultures. and are thus calculated for the period of semi-continuous cultures. rdiatom (d–1) and Kdiatom (104 cells ml–1) are the maximum growth rate and carrying capacity for P. tricornutum in monoculture, and rdino and Kdino for P. minimum (Supplementary Table 1). In this model, α and β are interaction coefficients, with α representing the impact of the dinoflagellates on the diatoms and β indicating that of the diatoms on the dinoflagellates. In this study, we focused on the impact of the dinoflagellates and the diatoms, as ocean-related global change generally enhances the competitive superiority of dinoflagellates over diatoms in many areas of the ocean (Morse et al., 2013; Xie et al., 2015; Carreto et al., 2018). Thus, we analyzed the responses of α. The dinoflagellates have the competitive superiority at α > 0; the diatoms have the competitive superiority at α < 0; and there is no competitive superiority for either species at α = 0.
Generalized linear mixed models (GLMMs) were used to investigate the factors determining phytoplankton competition, stoichiometric and lipid biomarker contents. Response variables were cell density, the interaction coefficient α, C:N:P stoichiometric molar ratios, FA biomarkers (16:1/16:0 and EPA/DHA), sterol biomarkers [the ratios of brassicasterol/epi-brassicasterol to (brassicasterol/epi-brassicasterol + dinosterol), B/(B + D)], with temperature, N:P supply ratios and nitrate concentrations as fixed effects. Target distributions were tested and link functions were consequently chosen. For all response variables, models containing first-order effects of the three factors, and second- and third-order interactions of all factors were tested. Model selection with the Akaike Information Criterion corrected (AICc) was used to determine the model that best predicted targets. A lower AICc value represents a better fit of the model, with 10 units or more difference being considered as a reasonable improvement in the models. If the difference in AICc values was smaller than 10 units, the simpler model was chosen, unless significant second- or third-order interactions were detected. Models containing only first-order effects of temperature, N:P supply ratios and nutrient concentrations were selected as the best models for interaction coefficient α, POC/PON, PON/POP, 16:1/16:0, and EPA/DHA, while that containing first-order effects and second-order interactions was chosen for B/(B + D) (Supplementary Table 2).
Generalized additive models (GAMs) were used to determine the amplitude of the response of the competition coefficient α to nitrate concentration under different temperatures, i.e., the difference between α at the strongest and the weakest effect of nitrate concentration under certain temperature condition. The GAMs were fitted separately for each of the three temperatures, as well as to the data from all temperature treatments. The partial additive effect of nitrate concentration on the coefficient α was visualized by GAM curves of partial residuals of the non-linear terms. The GAM results show that the individual temperature models explained 34.3–73.5% of the deviance in α, while the joined model with three temperatures explained 33.5% of the deviance (Table 2). Also, r2 and generalized cross-validation (GCV)-values were better in the individual temperature models than the joined model in most cases. Thus, the individual temperature models were selected, from which GAM curves of partial residuals of the non-linear estimation are presented in our study.
Linear regressions were conducted to test the relationship between PON/POP (and 16:1/16:0 and EPA/DHA) and the cell ratios of diatoms to dinoflagellates. Also, the correlations between B/(B + D) and the cell ratios of diatoms to the sum of diatoms and dinoflagellates were analyzed.
GLMMs and linear regressions were conducted in SPSS 19.0 (IBM Corporation). GAM was conducted using the “gam” function from the package mgcv (Wood, 2017) in R version 3.5.1 (R Development Core Team, 2010). Significance level was set to p < 0.05 in all statistical tests.
Results
Diatom-Dinoflagellate Competition Response
Dinoflagellates had the competitive superiority (α > 0) under low nutrient concentrations, but diatoms had the competitive superiority (α < 0) under high nutrient concentrations (Figure 2). The interaction coefficient α responded significantly to nutrient concentration changes (GLMMs; Table 3), showing a decrease as nutrient concentrations increased (Figure 2), while its responses to temperature and N:P supply ratios were not significant.
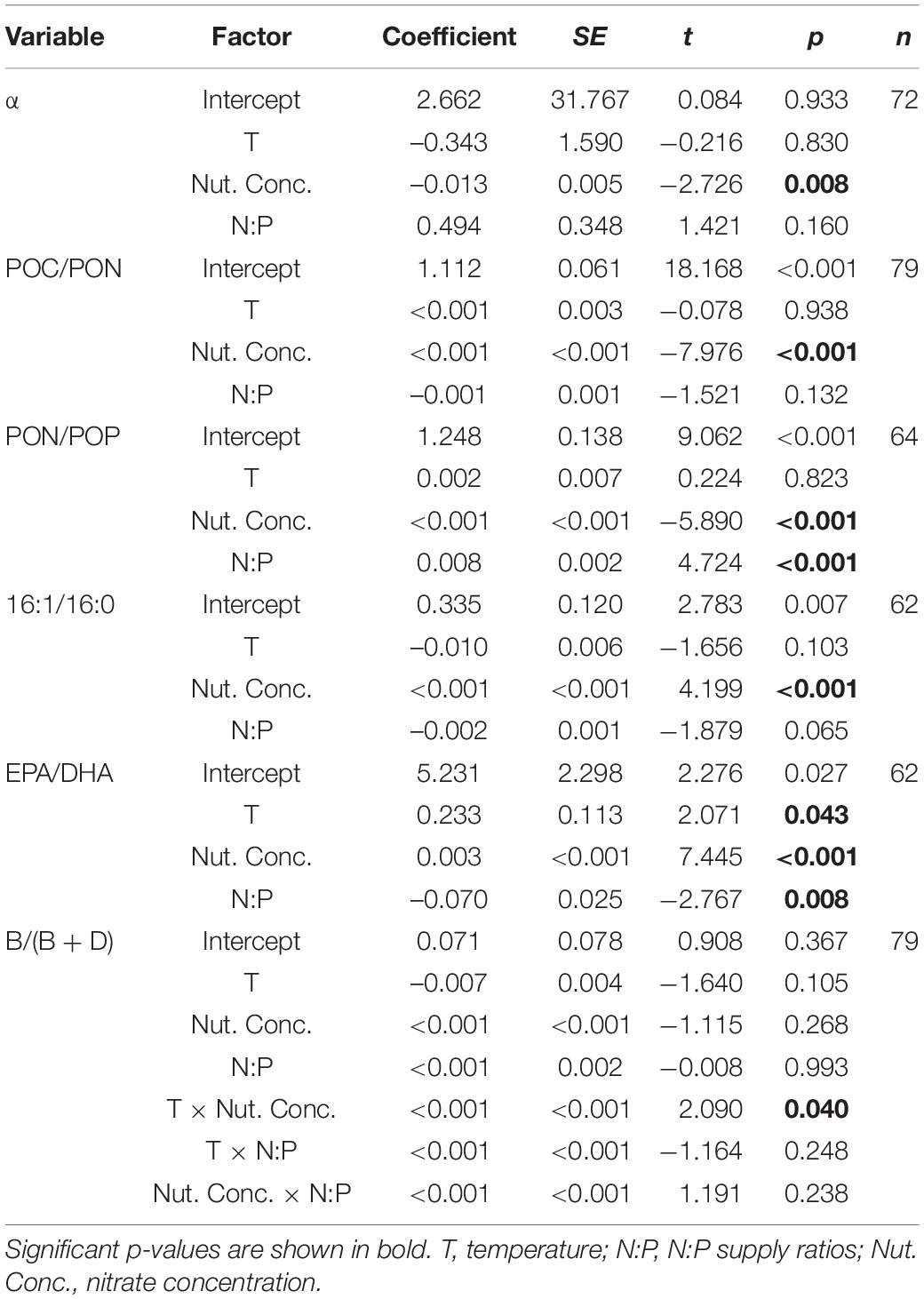
Table 3. Results of the selected GLMMs testing for the effects of temperature, N:P supply ratios, and nitrate concentrations on interaction coefficient α, POC/PON, PON/POP, 16:1/16:0, EPA/DHA and the ratios of brassicasterol/epi-brassicasterol to (brassicasterol/epi-brassicasterol + dinosterol) [B/(B + D)] in bicultures.
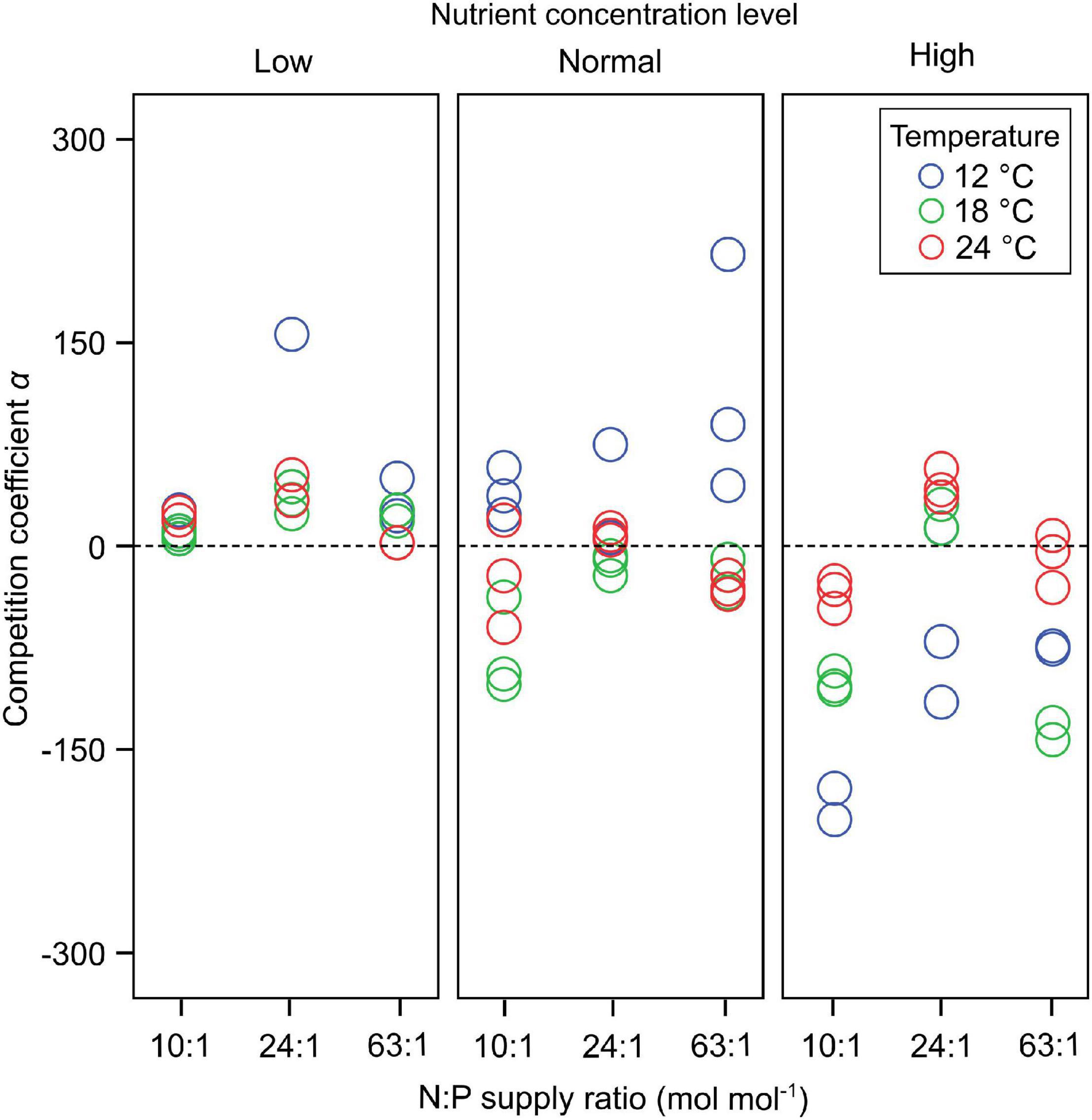
Figure 2. The responses of the interaction coefficient α to temperature, N:P supply ratios and nutrient concentrations in the bicultures of Phaeodactylum tricornutum and Prorocentrum minimum. At α > 0 dinoflagellates have the competitive superiority, while diatoms have the competitive superiority at α < 0. There is no competitive superiority for either species at α = 0.
The response amplitude of the competition coefficient α to nitrate concentration decreased with increasing temperature (Figure 3). The y-axis of Figure 3 indicates the partial additive effect of nitrate concentration on interaction coefficient α, with s () as the shorthand for fitting smoothing splines. While temperature per se had no overall effect on coefficient response, it was the amplitude of the response that was affected, not the direct response. Indeed, we observed that the interaction coefficient α also varied with temperature, showing higher values at the lowest temperature under normal-nutrient conditions, but higher values at higher temperatures under the highest nutrient concentrations (α > 0 indicating the competitive superiority of dinoflagellates; Figure 2).
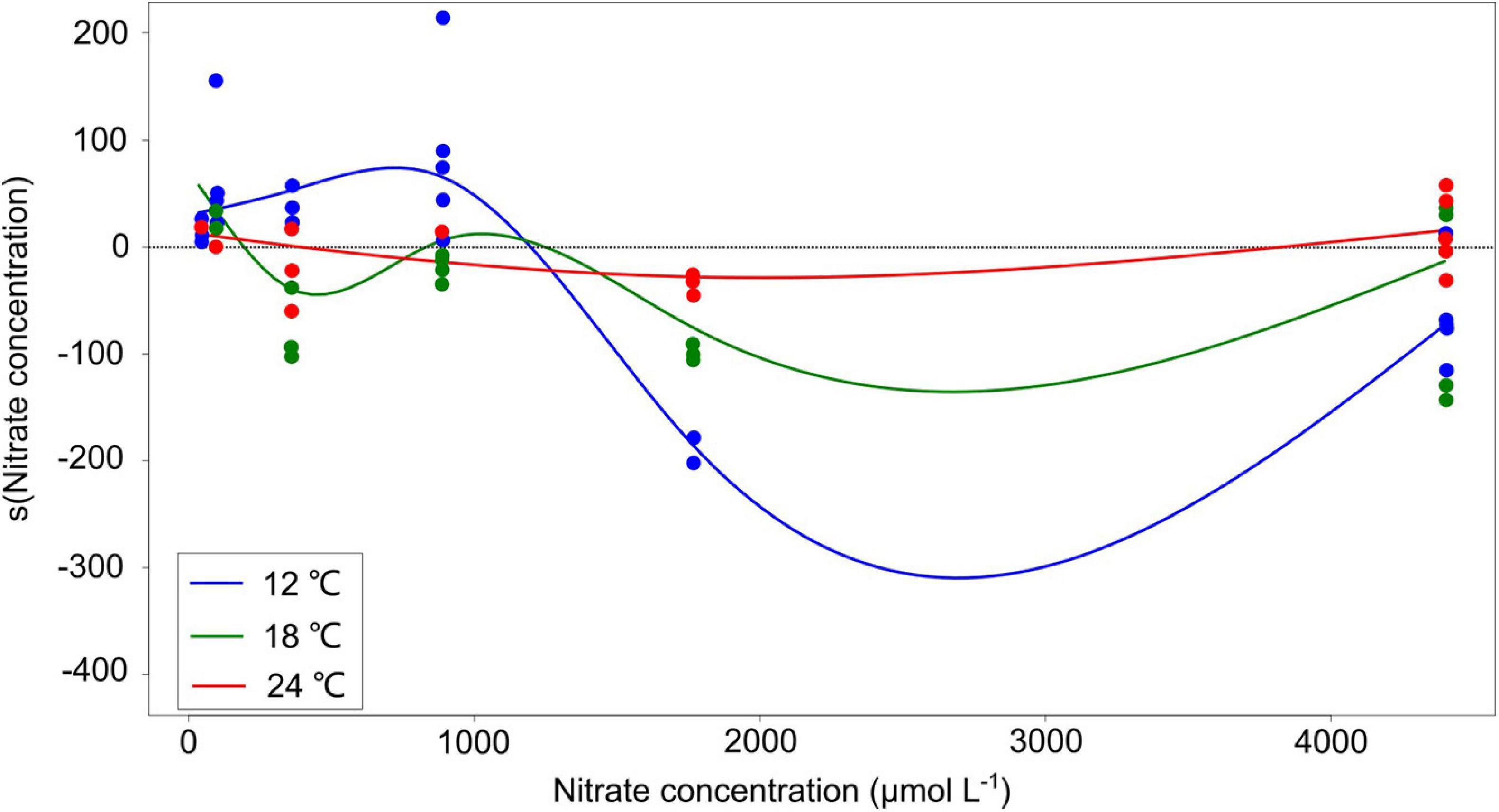
Figure 3. Partial residuals of the non-linear estimation from the generalized additive models (GAMs) with smoothing splines. The y-axis indicates the partial additive effect of nitrate concentration on interaction coefficient α, and s () is the shorthand for fitting smoothing splines. The solid curves are the estimates of the smooth with GAMs, and the dots represent observed data points. The summary of GAM results is shown in Table 2.
C:N:P Stoichiometry of Particulate Organic Matter
POC/PON responded significantly to changes in nutrient concentrations, but not to temperature or N:P supply ratios (GLMMs; Table 3 and Supplementary Table 1). POC/PON decreased with increasing nutrient concentrations (Figure 4A).
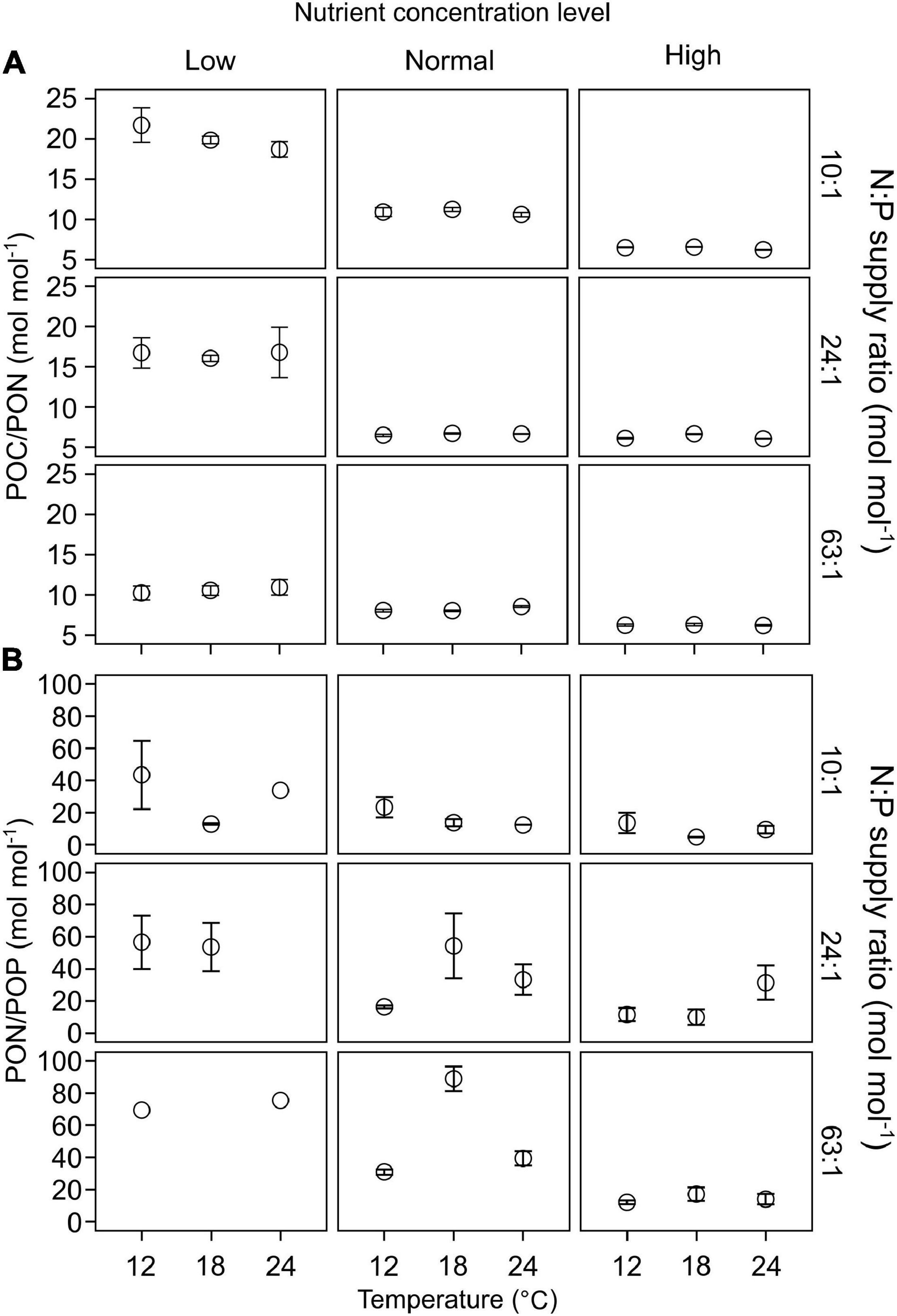
Figure 4. The responses of C:N:P stoichiometry in particulate organic matters (mean ± SE) to temperature, N:P supply ratios and nutrient concentrations in the bicultures of Phaeodactylum tricornutum and Prorocentrum minimum: (A) POC/PON and (B) PON/POP.
PON/POP responded significantly to nutrient concentrations and N:P supply ratios, but not to temperature (GLMMs; Table 3), showing general higher values at lower nutrient concentrations and higher N:P supply ratios (Figure 4B and Supplementary Figure 2). PON/POP showed a significant negative correlation with increasing diatoms/dinoflagellates cell ratios (Linear regression, p = 0.001; Figure 5A).
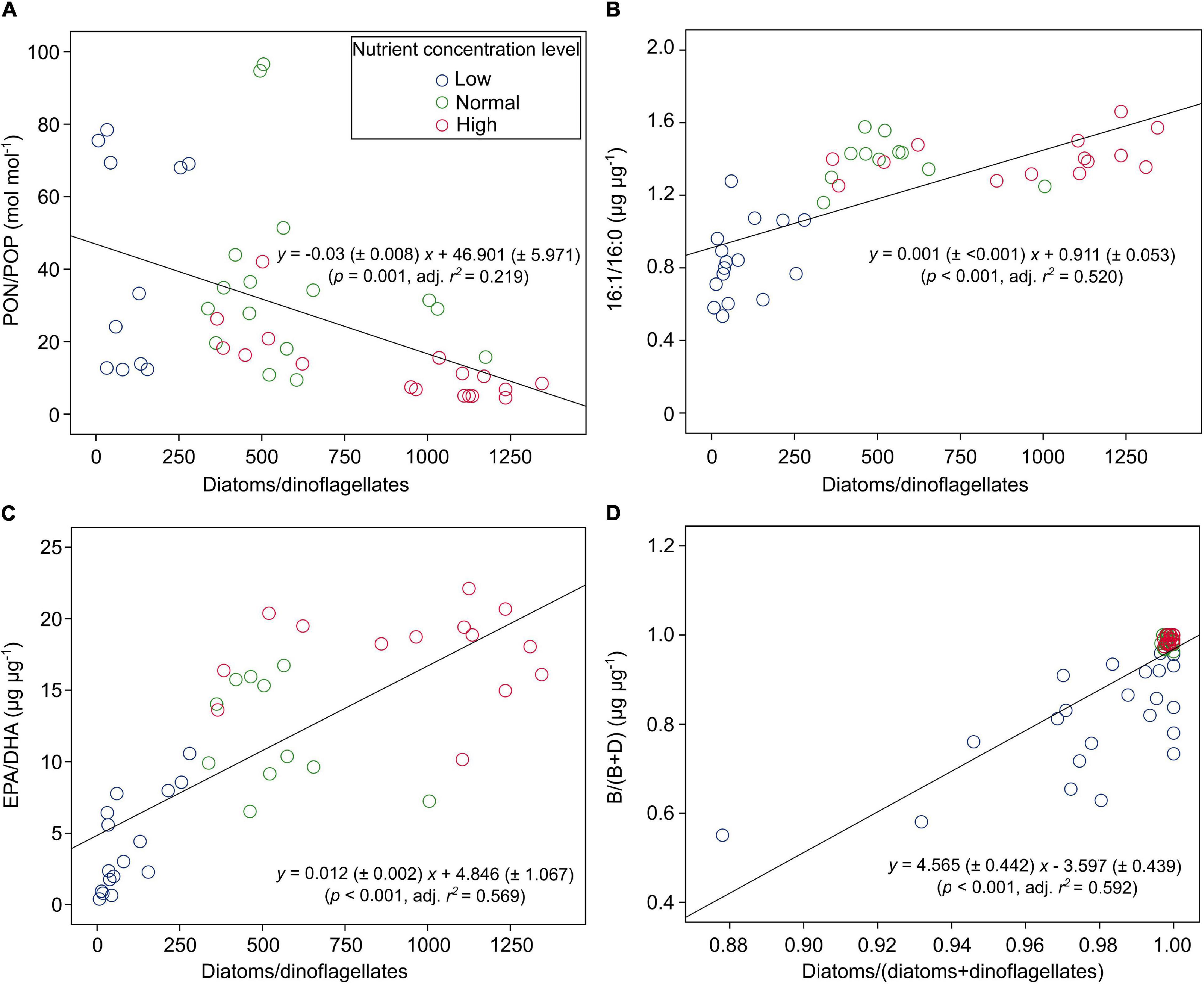
Figure 5. The correlations between PON/POP (and lipid biomarker ratios) and cell density ratios of diatoms/dinoflagellates [or diatoms/(diatoms + dinoflagellates)] under three levels of nutrient concentrations over the entire ranges of temperature and N:P supply ratios in the bicultures of Phaeodactylum tricornutum and Prorocentrum minimum: (A) PON/POP, (B) 16:1/16:0, (C) EPA/DHA, and (D) the ratios of brassicasterol/epi-brassicasterol to (brassicasterol/epi-brassicasterol + dinosterol) [B/(B + D)].
Lipid Biomarkers—Fatty Acids and Sterols
Of the 22 FA components identified in our study, seven contributed largely to TFAs (>5% of TFAs) over the entire ranges of temperature, N:P supply ratios and nutrient concentration levels (Supplementary Table 3). These included the saturated 14:0 and 16:0, monounsaturated 16:1n-7 and 18:1n-9c, and polyunsaturated 18:2n-6c, EPA and DHA. The ratios of both 16:1n-7 to 16:0 (16:1/16:0) and EPA to DHA (EPA/DHA) responded significantly to the changes in nutrient concentrations (GLMMs; Table 3), showing an increase with increasing nutrient concentrations (Figures 6A,B). Moreover, EPA/DHA also changed significantly with temperature and N:P supply ratios, showing overall higher values at higher temperatures and lower N:P ratios. 16:1/16:0 and EPA/DHA correlated positively with the cell density ratios of diatoms/dinoflagellates (Linear regression, p < 0.001; Figures 5B,C).
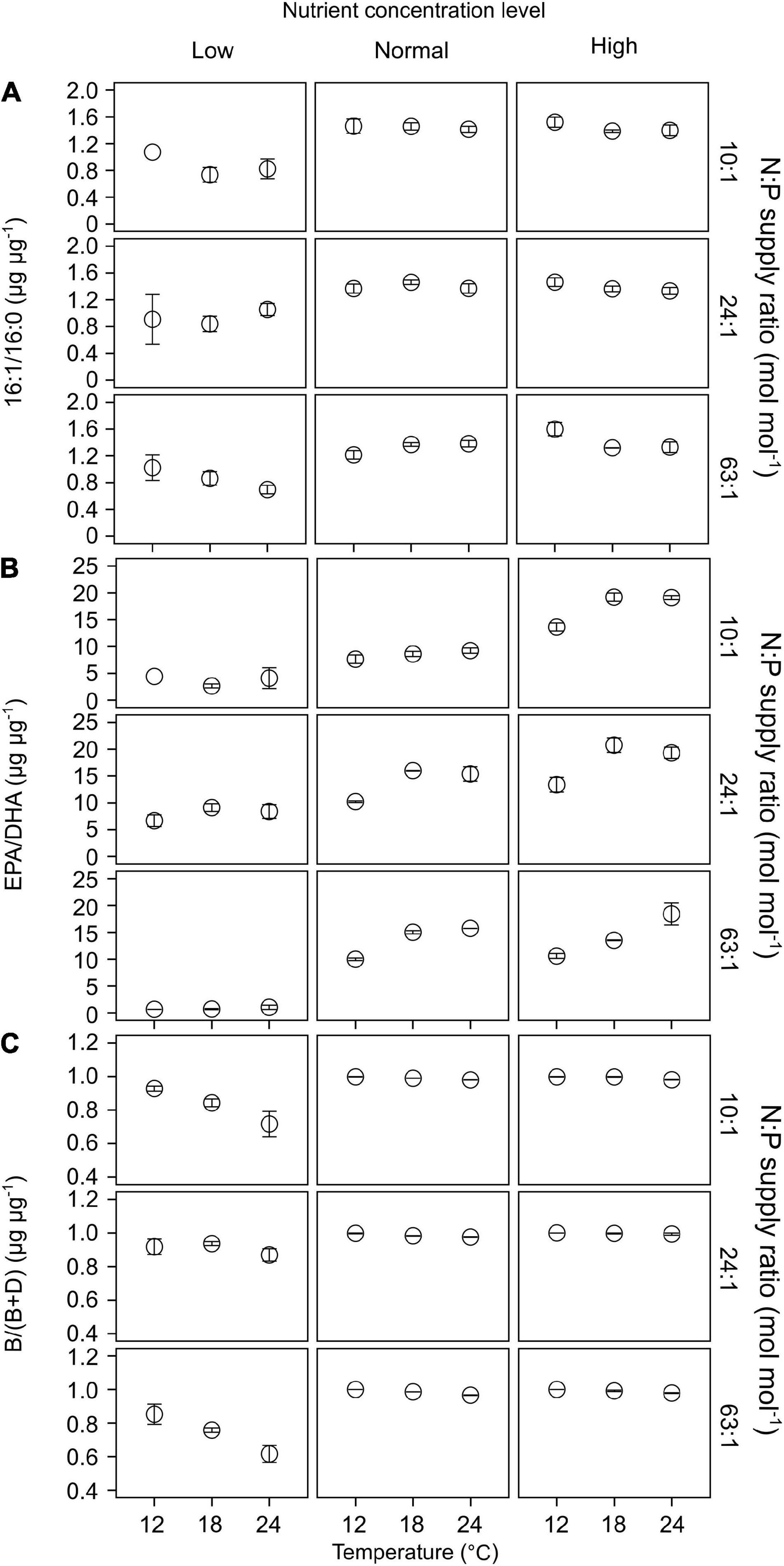
Figure 6. The responses of lipid biomarker ratios (mean ± SE) to temperature, N:P supply ratios and nutrient concentrations in the bicultures of Phaeodactylum tricornutum and Prorocentrum minimum: (A) 16:1/16:0, (B) EPA/DHA, and (C) the ratios of brassicasterol/epi-brassicasterol to (brassicasterol/epi-brassicasterol + dinosterol) [B/(B + D)].
Brassicasterol and dinosterol were two major sterols detected in bicultures (Supplementary Table 3). The ratios of brassicasterol/epi-brassicasterol to (brassicasterol/epi-brassicasterol + dinosterol) [B/(B + D)] responded significantly to the interactions of temperature and nutrient concentrations (GLMMs; Table 3), showing a decrease with increasing temperature at the lowest nutrient concentration (Figure 6C). B/(B + D) showed significant positive correlations with the cell density ratios of diatoms to the sum of diatoms and dinoflagellates (Linear regression, p < 0.001; Figure 5D).
Discussion
This study shows that the effect of nutrient concentrations is key in regulating diatom-dinoflagellate competition, as revealed by the competition coefficient, elemental stoichiometry and lipid biomarkers (FAs and sterols). Significant effects of temperature and N:P supply ratios were only observed on certain competition indices. Specifically, our results show competitive superiority of the diatom P. tricornutum over the dinoflagellate P. minimum at high nutrient concentrations, which holds independently of temperature conditions and N:P supply ratios in the culture medium. Although temperature showed non-significant effects on the competition coefficient, the variation in temperature altered the response amplitude of diatom-dinoflagellate competition to nutrient concentration changes, showing the interaction between the two environmental factors, consistent with previous findings in eutrophic coastal areas (Xiao et al., 2018). The changes in temperature and N:P supply ratios had significant effects on elemental and lipid composition in the diatom-dinoflagellate community, e.g., the increase in N:P ratios causing significant higher PON/POP at the two low nutrient levels. Such variations in chemical composition of the diatom-dinoflagellate community significantly correlated with the changes in the relative abundance of diatoms and dinoflagellates.
Diatom-Dinoflagellate Competition Under Different Temperature and Nutrient Regimes
Nutrient concentrations had significant effects on the interaction coefficient α of the diatom-dinoflagellate competition (Table 3). Specifically, the responses of the interaction coefficient α to nitrate concentration reveal two scenarios. On the one hand, interaction coefficient α showed a switch from a competitive superiority of the dinoflagellates to the diatoms as nitrate concentration increased at the low nutrient regime (Figure 3), indicating that the dinoflagellates favor low nutrient condition, but the diatoms favor high nutrients. Our results are consistent with the succession trends in phytoplankton communities in the world ocean where high concentrations of nitrate are encountered, mainly in highly eutrophic bays and river inlets (Aubry et al., 2004; Gettings et al., 2014; Mutshinda et al., 2016; Barcelos e Ramos et al., 2017; Ratmaya et al., 2019). For example, the Bay of Brest received high-nitrate-loaded freshwater (>700 μmol L–1) in winter in 1990s, which supported a diatom-dominated spring bloom (Del Amo et al., 1997). Comparable situations have been reported for the Changjiang Estuary and adjacent shelf of the ECS and southern Yellow Sea, where diatoms dominated within river plume and high-DIN regions (up to 50 μmol L–1), while the density of dinoflagellates was typically high at low-nutrient areas outsides diatom-blooming zones (Jiang et al., 2015, 2019). The diatom-dinoflagellate succession along nitrate concentration changes aligns with ecological traits defined by Margalef (1978). The diatom P. tricornutum appeared to have high requirements for nutrients (r-strategy), and the dinoflagellate P. minimum outcompeted the diatoms at low nutrient conditions (K-strategy). Genetic analysis has shown the remodeling of intermediate metabolism in P. tricornutum, allowing the diatoms to efficiently respond to changes in nitrogen availability and thereby to become ecologically successful in the aquatic environments where nitrogen can be introduced rapidly through turbulence (Levitan et al., 2015; Smith et al., 2019). In contrast, dinoflagellate abundance has shown a positive correlation with N-acquisition genes, which is linked to the competitive advantage of dinoflagellates at low nutrient conditions (Zhuang et al., 2015; Elferink et al., 2020).
On the other hand, the response amplitude of the interaction coefficient α to nitrate concentration shows a switch back toward competitive superiority of the dinoflagellates at the high nitrate regime (Figure 3). Note that only at the highest temperature (24°C) and only at the very high nitrate concentrations, the partial residuals of the non-linear terms in the GAM plot truly became positive again (the red curve in Figure 3). The results are in agreement with field observations, which show that climate warming and high nutrient concentration play a dominant role in the phytoplankton assemblage changing from the diatoms to the dinoflagellates in different regions, e.g., the Chesapeake Bay (Malone, 1992), the north Atlantic (Leterme et al., 2005), the western English Channel (Xie et al., 2015), and the Baltic Sea (Spilling et al., 2018). In particular, the extent of the increase in nutrient concentrations in our study (5-fold higher than f/2 medium) is comparable with that during 2002–2015 in the ECS (∼5- to 10-fold increase; NO2– + NO3–: between 0–1.0 and 10–50 μmol L–1; PO43–: between 0–0.08 and 0.8–1.5 μmol L–1) (Xiao et al., 2018). Consistent with our study, model simulations on the diatom-dinoflagellate competition demonstrated that the spring dinoflagellate bloom in the coastal ECS is projected to increase at high temperature (>∼24°C) and high nutrient concentrations (PO43–: 0.25 and 0.6 μmol L–1, ∼5- to 10-fold more than the lowest concentration 0.05 μmol L–1) (Xiao et al., 2018). An explanation for the switch back toward competitive superiority of the dinoflagellates is that the highest temperature 24°C in our study is above the temperature optimum (around 20°C) for the growth of P. tricornutum (William and Morris, 1982), while it is within the higher optimal temperatures for P. minimum (around 18–26°C) (Grzebyk and Berland, 1996). Furthermore, P. minimum can also form toxic blooms, particularly occurred under warm and eutrophic coastal waters in subtropical and temperate climatic zones (Heil et al., 2005). It has been observed that allelochemicals produced by P. minimum significantly inhibited the growth of the diatom Skeletonema costatum (Tameishi et al., 2009). For another dinoflagellate Prorocentrum lima, increasing temperature may lead to higher cellular toxin levels (Aquino-Cruz et al., 2018). Therefore, the allelopathic effects of P. minimum on P. tricornutum may be partially attributed to the competitive dominance of P. minimum at the highest temperature and nitrate concentrations in our study, which should be tested in further studies.
In summary, we observed a switch from a competitive superiority of the dinoflagellates to the diatoms with increasing nutrient concentrations, and the shift back to dinoflagellates at very high nutrient levels and high temperatures. While the former responses in the diatom-dinoflagellate competition have been previously observed, the latter has not been even covered in classical competition theories, which focused on resource competition and were not coupled with increasing temperature (Tilman, 1982; Sommer, 1989; Smayda and Reynolds, 2003). Our results reveal strong impacts of temperature and nutrient concentrations on diatom-dinoflagellate competition, which are consistent with previous studies, indicating that dinoflagellates are more competitive than diatoms and often form harmful algal blooms under high nutrients and warming conditions, particularly in highly eutrophic bays and coastal areas (Xiao et al., 2018; Glibert, 2020).
Stoichiometric C:N:P and Diatom-Dinoflagellate Competition
We found significant responses of POC/PON and PON/POP to the changes in nutrient concentrations and N:P supply ratios (only for PON/POP), showing lower values at higher nutrient concentrations (Table 3 and Figure 4), but there were no significant responses to temperature changes. In support, a recent meta-analysis demonstrated that the availability of dissolved inorganic phosphorus and nitrogen is the most significant and shared environmental driver of phytoplankton C:N:P, with POP:POC and PON:POC positively related to the increased in nutrient availability (Tanioka and Matsumoto, 2020). These results are consistent with the nutrient supply hypothesis, which predicts the dependence of ecosystem stoichiometric flexibility on dissolved nutrient concentrations (Galbraith and Martiny, 2015; Garcia et al., 2018). Contrary to our findings, the effects of temperature on phytoplankton stoichiometric variation has been also observed (Toseland et al., 2013; Yvon-Durocher et al., 2015; Bi et al., 2017). For example, the optimal N:P (i.e., threshold N:P at which N and P are co-limiting) is temperature dependent in the green alga Chlamydomonas reinhardtii (Thrane et al., 2017). Because nutrient availability can change the extent or even reverse the effects of temperature on phytoplankton C:N:P stoichiometry (Bi et al., 2017), the effects of temperature may be more pronounced under certain nutrient conditions. Nevertheless, our results illustrate that nutrient concentrations highly regulate stoichiometric C:N:P in the diatom-dinoflagellate bicultures.
Furthermore, PON/POP correlated negatively with cell density ratios of diatoms/dinoflagellates in our study (Figure 5A). In line with previous studies (e.g., Weber and Deutsch, 2010; Martiny et al., 2013; Martiny et al., 2016), our results indicate the link between elemental stoichiometry and phytoplankton community structures, in this case, reduced PON/POP was associated with an elevated contribution of diatoms to the diatom-dinoflagellate bicultures. This linkage can be explained by phytoplankton group-specific C:N:P stoichiometry, i.e., lower PON/POP in diatoms than other phytoplankton groups (Quigg et al., 2003; Weber and Deutsch, 2010; Sharoni and Halevy, 2020). Differences in stoichiometric C:N:P composition between phytoplankton groups reflect the variations in biochemical composition, such as protein, ribosomal RNA and lipids (Geider and La Roche, 2002; Sterner and Elser, 2002; Loladze and Elser, 2011; Bi et al., 2014), and in luxury consumption of nutrients, e.g., the accumulation of phosphorus storage pools like polyphosphate (Sterner and Elser, 2002; Martin et al., 2014; Lin et al., 2016). It has been found that residual P (intracellular storage or surface adsorption of inorganic P) rather than nucleic acids and phospholipids dominates the variability in phytoplankton PON/POP during N starvation, with more than 80% of cellular P allocated to residual P in diatoms and only 60% in prasinophytes (Liefer et al., 2019). Such mechanistic insights of residual P pools may therefore indicate a greater luxury uptake of P in diatoms than dinoflagellates, especially under high nutrient concentrations in our study, where diatoms became dominant associated with low PON/POP.
Overall, our study shows that stoichiometric C:N:P varied significantly with changes in N and P concentrations, indicating the fundamental roles of nutrient availability in phytoplankton stoichiometry. Also, PON/POP responded positively to increasing N:P supply ratios, which was more pronounced at low levels of N and P (≤the concentrations in the commonly used f/2 medium) (Supplementary Figure 2). The optimal allocation strategy of N and P in cellular machinery may decouple PON/POP from N:P supply ratios at the highest nutrient level in our study (Klausmeier et al., 2004). Moreover, we found that C:N:P stoichiometry significantly correlated with the shift in the diatom-dinoflagellate community, revealing important implications for marine biogeochemistry and food web dynamics. For example, the competitive superiority of diatoms with low PON/POP can reduce the phosphorus available for nitrogen fixation, with the opposite situation for the competitive superiority of dinoflagellates (Mills and Arrigo, 2010). The relatively low POC/PON at the competitive superiority of the diatoms represents a high elemental quality of food for zooplankton, as high N-rich compounds promote the growth and reproduction of consumers; in contrast, the high POC/PON at the competitive superiority of the dinoflagellates reveals an inferior quality of food (Anderson et al., 2004). The low elemental quality of food may reduce the production of higher trophic levels. An example is the long-term change in the copepod communities in the southern German Bight, where the decrease in elemental food quality of phytoplankton has been suggested to cause a strong decrease in calanoid copepod densities and a decline in herring recruitment during 1975–2011 (Boersma et al., 2015).
Lipid Biomarkers and Diatom-Dinoflagellate Competition
FA Biomarkers
In our study, the two FA biomarkers, 16:1/16:0 and EPA/DHA, had highly significant responses to the changes in nutrient concentrations, showing higher values at higher nutrient concentrations (Table 3 and Figures 6A,B). Similarly, the interaction coefficient α also responded significantly to nutrient concentrations in our study. Indeed, the two FA biomarkers correlated positively with cell density ratios of diatoms/dinoflagellates (Figures 5B,C). Field and laboratory studies have shown that high values of 16:1/16:0 and EPA/DHA can indicate the dominance of diatoms vs. dinoflagellates in phytoplankton communities (Reuss and Poulsen, 2002; Cañavate, 2019; Kohlbach et al., 2021). Our data add to previous findings, suggesting a strong correlation between FA composition and phytoplankton community structure over large temperature and nutrient ranges (Dalsgaard et al., 2003; Galloway and Winder, 2015).
Furthermore, we found that EPA/DHA responded significantly to temperature and N:P supply ratios (Table 3), being higher at higher temperatures and lower N:P supply ratios under high nutrient regimes (Figure 6B); however, the interaction coefficient α showed non-significant responses to temperature or N:P supply ratios. As the diatoms were overall dominant at higher nutrient concentrations, the observed changes in EPA/DHA preliminarily reflected the responses of diatom FAs to environmental changes. On top of phylogeny, environmental conditions can also determine phytoplankton FA composition (reviwed by Dalsgaard et al., 2003; Galloway and Winder, 2015; Jónasdóttir, 2019). Previous field studies reported that the main driver of FA composition of phytoplankton was environmental changes, such as seasonal fluctuations in freshwater inflow in San Francisco Bay (Canuel, 2001), and nutrient availability in coastal areas of the Western English Channel (White et al., 2015). Trommer et al. (2019) reported that the shifts in PUFA composition in lake Brunnensee in Germany were independent of algal group succession, but was rather dependent on the changes in per-cell PUFA contents in phytoplankton in response to an increase in nitrogen supply. Our laboratory experiments further quantified the relationship between FA markers and cell density ratios of diatoms/dinoflagellates, showing that the relationship between EPA/DHA and diatoms/dinoflagellates was highly discrete at the highest nutrient concentration (Figure 5C), which can be attributed to the significant effects of temperature and N:P supply ratios (Table 3 and Figure 6B). Our results thus suggest that the effects of environmental factors on phytoplankton FA composition can to some extent modify the relationship between FA biomarkers and phytoplankton community structure.
Sterol Biomarkers
The ratios of brassicasterol/epi-brassicasterol to (brassicasterol/epi-brassicasterol + dinosterol) [B/(B + D)] responded significantly to the interactions between temperature and nutrient concentrations, showing a clear decrease with increasing temperature at the lowest nutrient concentration (Figure 6C). Such a decrease in B/(B + D) was associated with a strong increase in carbon-normalized dinosterol contents, but no clear change in brassicasterol/epi-brassicasterol contents (Supplementary Figure 3), in agreement with the competitive superiority of dinoflagellates at the lowest nutrient concentration (Figure 2).
We further showed that B/(B + D) had a good correlation with cell density ratios of diatoms to the sum of two species density (Figure 5D). Our laboratory results are consistent with those in field studies, which have shown the applicability of brassicasterol and dinosterol as proxies of community structure of diatoms and dinoflagellates in suspended particles, e.g., in the Crozet plateau, Southern Ocean (Hernandez et al., 2008), the West Pacific (Dong et al., 2012), and the ECS (Wu et al., 2016; Bi et al., 2018). The present study provides strong evidence for the application of brassicasterol and dinosterol to reveal community structures of diatoms and dinoflagellates under highly variable environmental conditions.
To summarize, our study shows that nutrient concentrations had significant effects on all biomarkers of FAs and sterols [16:1/16:0, EPA/DHA and B/(B + D)], while temperature and N:P supply ratios showed a significant influence only on certain biomarker indices. The variations in lipid biomarkers were overall consistent with those in cell density ratios between diatoms to dinoflagellates. Our results reveal strong ecological relevance for the understanding of the roles of lipid biomarkers in food webs. EPA and DHA are particularly important for animals to meet their needs for population growth and reproduction (Arts et al., 2001; Arendt et al., 2005; Jónasdóttir et al., 2009; Malzahn and Boersma, 2012). These two essential FAs play important but different ecological roles in food webs, showing different distribution patterns within food webs, e.g., EPA highly retained in zooplankton but DHA in fish (Kainz et al., 2004). It has been recently observed that flagellate-dominated phytoplankton communities in summer conferred a higher nutritional value, i.e., higher DHA/EPA ratio, compared to diatom-dominated community in spring, indicating a better quality of zooplankton for fish in summer in the Strait of Georgia in northeast Pacific (Costalago et al., 2020). Nevertheless, our results show significant correlations between lipid biomarkers and diatom-dinoflagellate community composition over wide ranges of temperature and nutrient conditions, indicating that the shift in diatom-dinoflagellate community can result in the fluctuations in the dietary lipids and consequently alter the production of higher trophic levels.
Conclusion
This study demonstrates that diatom-dinoflagellate competition responded strongly to the changes in nutrient concentrations, while the amplitude of this response was modified by temperature. Specifically, our results show a switch from a competitive superiority of dinoflagellates to diatoms with increasing nitrate concentrations at the low nutrient regime, and a switch back toward the competitive superiority of the dinoflagellates at the high nitrate regime and the highest temperature. These results are consistent with previous field observations, suggesting that dinoflagellates are more competitive than diatoms under high nutrients and warming conditions, particularly in highly eutrophic bays and coasts. Our findings present an important step to quantitatively assess the effects of multiple environmental drivers on diatom-dinoflagellate competition.
Furthermore, elemental stoichiometry and lipid biomarkers in bicultures changed significantly with nutrient concentrations, but significant effects of temperature and N:P supply ratios were only observed on certain parameters. We quantified the relationship between cell density ratios of the two species and their chemical composition in bicultures, showing that the shift in the diatom-dinoflagellate community was significantly correlated with the fluctuations in elemental and biochemical composition over wide ranges of temperature, N and P concentrations and N:P ratios studied in this work. The variations in elemental and biochemical composition reveal a potential far-reaching consequence of the diatom-dinoflagellate succession on biogeochemical cycles and ecological function, e.g., influencing the process of nitrogen fixation, and changing nutritional quality of phytoplankton for higher trophic levels. In addition to the importance of species-specific responses in phytoplankton which is not the focus of this study, our results based on two typical species are very consistent with field observations and ecological modelings. Future work should thoroughly focus on other species and more complex phytoplankton communities to refine our understanding of biogeochemical and ecological consequences of the diatom-dinoflagellate competition.
Data Availability Statement
The raw data supporting the conclusions of this article will be made available by the authors, without undue reservation. Data supporting the conclusions are also publicly available at https://doi.org/10.5281/zenodo.5205293.
Author Contributions
RB and MZ designed the study. RB and ZC performed the experiments, with assistance of HZ and YD. SI-R and US provided comments on the data analysis. RB wrote the manuscript with contributions from all co-authors. All authors contributed to the article and approved the submitted version.
Funding
This study was supported by the National Natural Science Foundation of China (Grant Nos. 41630966 and 41876118), the Youth Talent Support Program of the Laboratory for Marine Ecology and Environmental Science, the Pilot National Laboratory for Marine Science and Technology (Qingdao) (Grant No. LMEES-YTSP-2018-02-01), and the GEOMAR Helmholtz-Zentrum für Ozeanforschung Kiel.
Conflict of Interest
The authors declare that the research was conducted in the absence of any commercial or financial relationships that could be construed as a potential conflict of interest.
Publisher’s Note
All claims expressed in this article are solely those of the authors and do not necessarily represent those of their affiliated organizations, or those of the publisher, the editors and the reviewers. Any product that may be evaluated in this article, or claim that may be made by its manufacturer, is not guaranteed or endorsed by the publisher.
Acknowledgments
We would like to thank Li Li, Guie Jin, Yu Zhan, and Xiaoke Xin for technical assistance, and Yaoyao Wang, Peng Peng, Jiawei Gao, and Chuanli Zhang for helping with the experiments. We are grateful to Xupeng Chi for his comments on the analysis of generalized additive models. We thank two reviewers for constructive comments. This is MCTL (Key Laboratory of Marine Chemistry Theory and Technology) contribution #259.
Supplementary Material
The Supplementary Material for this article can be found online at: https://www.frontiersin.org/articles/10.3389/fmicb.2021.731786/full#supplementary-material
References
Anderson, T. R., Boersma, M., and Raubenheimer, D. (2004). Stoichiometry: linking elements to biochemicals. Ecology 85, 1193–1202. doi: 10.1890/02-0252
Aquino-Cruz, A., Purdie, D. A., and Morris, S. (2018). Effect of increasing sea water temperature on the growth and toxin production of the benthic dinoflagellate Prorocentrum lima. Hydrobiologia 813, 103–122. doi: 10.1007/s10750-018-3512-4
Arendt, K. E., Jónasdóttir, S. H., Hansen, P. J., and Gärtner, S. (2005). Effects of dietary fatty acids on the reproductive success of the calanoid copepod Temora longicornis. Mar. Biol. 146, 513–530. doi: 10.1007/s00227-004-1457-9
Armbrust, E. V., Berges, J. A., Bowler, C., Green, B. R., Martinez, D., Putnam, N. H., et al. (2004). The genome of the diatom Thalassiosira pseudonana: ecology, evolution, and metabolism. Science 306, 79–86. doi: 10.1126/science.1101156
Arts, M. T., Ackman, R. G., and Holub, B. J. (2001). “Essential fatty acids” in aquatic ecosystems: a crucial link between diet and human health and evolution. Can. J. Fish. Aquat. Sci. 58, 122–137. doi: 10.1139/f00-224
Aubry, F. B., Berton, A., Bastianini, M., Socal, G., and Acri, F. (2004). Phytoplankton succession in a coastal area of the NW Adriatic, over a 10-year sampling period (1990-1999). Cont. Shelf Res. 24, 97–115. doi: 10.1016/j.csr.2003.09.007
Bach, L. T., Paul, A. J., Boxhammer, T., von der Esch, E., Graco, M., Schulz, K. G., et al. (2020). Factors controlling plankton community production, export flux, and particulate matter stoichiometry in the coastal upwelling system off Peru. Biogeosciences 17, 4831–4852. doi: 10.5194/bg-17-4831-2020
Barcelos e Ramos, J., Schulz, K. G., Voss, M., Narciso, A., Mueller, M. N., Reis, F. V., et al. (2017). Nutrient-specific responses of a phytoplankton community: a case study of the North Atlantic Gyre, Azores. J. Plankton Res. 39, 744–761. doi: 10.1093/plankt/fbx025
Beardall, J., and Morris, I. (1976). The concept of light intensity adaptation in marine phytoplankton: some experiments with Phaeodactylum tricornutum. Mar. Biol. 37, 377–387. doi: 10.1007/BF00387494
Bi, R., Arndt, C., and Sommer, U. (2014). Linking elements to biochemicals: effects of nutrient supply ratios and growth rates on fatty acid composition of phytoplankton species. J. Phycol. 50, 117–130. doi: 10.1111/jpy.12140
Bi, R., Chen, X., Zhang, J., Ishizaka, J., Zhuang, Y., Jin, H., et al. (2018). Water mass control on phytoplankton spatiotemporal variations in the northeastern East China Sea and the western Tsushima Strait revealed by lipid biomarkers. J. Geophys. Res. Biogeosci. 123, 1318–1332. doi: 10.1002/2017JG004340
Bi, R., Ismar, S. M. H., Sommer, U., and Zhao, M. (2017). Environmental dependence of the correlations between stoichiometric and fatty acid-based indicators of phytoplankton nutritional quality. Limnol. Oceanogr. 62, 334–347. doi: 10.1002/lno.10429
Bi, R., Ismar-Rebitz, S. M. H., Sommer, U., Zhang, H., and Zhao, M. (2020). Ocean-related global change alters lipid biomarker production in common marine phytoplankton. Biogeosciences 17, 6287–6307. doi: 10.5194/bg-17-6287-2020
Bi, R., and Sommer, U. (2020). Food quantity and quality interactions at phytoplankton–zooplankton interface: chemical and reproductive responses in a calanoid copepod. Front. Mar. Sci. 7:274. doi: 10.3389/fmars.2020.00274
Bindoff, N. L., Cheung, W. W. L., Kairo, J. G., Arístegui, J., Guinder, V. A., Hallberg, R., et al. (2019). “Changing ocean, marine ecosystems, and dependent communities,” in IPCC Special Report on the Ocean and Cryosphere in a Changing Climate, eds H.-O. Pörtner, D. C. Roberts, V. Masson-Delmotte, P. Zhai, M. Tignor, E. Poloczanska, et al. (Geneva: IPCC).
Boersma, M., Wiltshire, K. H., Kong, S.-M., Greve, W., and Renz, J. (2015). Long-term change in the copepod community in the southern German Bight. J. Sea Res. 101, 41–50. doi: 10.1016/j.seares.2014.12.004
Bojko, M., Brzostowska, K., Kuczynska, P., Latowski, D., Olchawa-Pajor, M., Krzeszowiec, W., et al. (2013). Temperature effect on growth, and selected parameters of Phaeodactylum tricornutum in batch cultures. Acta Biochim. Pol. 60, 861–864.
Bonnet, S., Guieu, C., Bruyant, F., Prasil, O., Van Wambeke, F., Raimbault, P., et al. (2008). Nutrient limitation of primary productivity in the Southeast Pacific (BIOSOPE cruise). Biogeosciences 5, 215–225. doi: 10.5194/bg-5-215-2008
Burkhardt, S., Zondervan, I., and Riebesell, U. (1999). Effect of CO2 concentration on C:N:P ratio in marine phytoplankton: a species comparison. Limnol. Oceanogr. 44, 683–690. doi: 10.4319/lo.1999.44.3.0683
Cañavate, J. P. (2019). Advancing assessment of marine phytoplankton community structure and nutritional value from fatty acid profiles of cultured microalgae. Rev. Aquat. Sci. 11, 527–549. doi: 10.1111/raq.12244
Canuel, E. A. (2001). Relations between river flow, primary production and fatty acid composition of particulate organic matter in San Francisco and Chesapeake Bays: a multivariate approach. Org. Geochem. 32, 563–583. doi: 10.1016/s0146-6380(00)00195-9
Carreto, J. I., Carignan, M. O., Montoya, N. G., Cozzolino, E., and Akselman, R. (2018). Mycosporine-like amino acids and xanthophyll-cycle pigments favour a massive spring bloom development of the dinoflagellate Prorocentrum minimum in Grande Bay (Argentina), an ozone hole affected area. J. Mar. Syst. 178, 15–28. doi: 10.1016/j.jmarsys.2017.10.004
Cloern, J. E., Abreu, P. C., Carstensen, J., Chauvaud, L., Elmgren, R., Grall, J., et al. (2016). Human activities and climate variability drive fast-paced change across the world’s estuarine-coastal ecosystems. Glob. Change Biol. 22, 513–529. doi: 10.1111/gcb.13059
Costalago, D., Forster, I., Nemcek, N., Neville, C., Perry, R. I., Young, K., et al. (2020). Seasonal and spatial dynamics of the planktonic trophic biomarkers in the Strait of Georgia (northeast Pacific) and implications for fish. Sci. Rep. 10:8517. doi: 10.1038/s41598-020-65557-1
Cross, W. F., Hood, J. M., Benstead, J. P., Huryn, A. D., and Nelson, D. (2015). Interactions between temperature and nutrients across levels of ecological organization. Glob. Change Biol. 21, 1025–1040. doi: 10.1111/gcb.12809
Dalsgaard, J., St. John, M., Kattner, G., Müller-Navarra, D., and Hagen, W. (2003). Fatty acid trophic markers in the pelagic marine environment. Adv. Mar. Biol. 46, 225–340. doi: 10.1016/S0065-2881(03)46005-7
Del Amo, Y., Le Pape, O., Treguer, P., Queguiner, B., Menesguen, A., and Aminot, A. (1997). Impacts of high-nitrate freshwater inputs on macrotidal ecosystems. I. Seasonal evolution of nutrient limitation for the diatom-dominated phytoplankton of the Bay of Brest (France). Mar. Ecol. Prog. Ser. 161, 213–224. doi: 10.3354/meps161213
Delwiche, C. F. (2007). “CHAPTER 10 - The origin and evolution of dinoflagellates,” in Evolution of Primary Producers in the Sea, eds P. G. Falkowski and A. H. Knoll (Burlington, VT: Academic Press), 191–205.
Ding, Y., Bi, R., Sachs, J. P., Chen, X., Zhang, H. L., Li, L., et al. (2019). Lipid biomarker production by marine phytoplankton under different nutrient and temperature regimes. Org. Geochem. 131, 34–49. doi: 10.1016/j.orggeochem.2019.01.008
Dong, L., Li, L., Wang, H., Hu, J., and Wei, Y. (2012). Phytoplankton distribution in surface water of Western Pacific during winter, 2008: a study of molecular organic geochemistry. Mar. Geol. Quat. Geol. 32, 51–59.
Elferink, S., John, U., Neuhaus, S., and Wohlrab, S. (2020). Functional genomics differentiate inherent and environmentally influenced traits in dinoflagellate and diatom communities. Microorganisms 8:567. doi: 10.3390/microorganisms8040567
Falkowski, P. G., and Oliver, M. J. (2007). Mix and match: how climate selects phytoplankton. Nat. Rev. Microbiol. 5, 813–819. doi: 10.1038/nrmicro1792
Fisher, N. L., Campbell, D. A., Hughes, D. J., Kuzhiumparambil, U., Halsey, K. H., Ralph, P. J., et al. (2020). Divergence of photosynthetic strategies amongst marine diatoms. PLoS One 15:e0244252. doi: 10.1371/journal.pone.0244252
Galbraith, E. D., and Martiny, A. C. (2015). A simple nutrient-dependence mechanism for predicting the stoichiometry of marine ecosystems. Proc. Natl. Acad. Sci. U.S.A. 112, 8199–8204. doi: 10.1073/pnas.1423917112
Galloway, A. W. E., and Winder, M. (2015). Partitioning the relative importance of phylogeny and environmental conditions on phytoplankton fatty acids. PLoS One 10:e0130053. doi: 10.1371/journal.pone.0130053
Gao, G., Shi, Q., Xu, Z., Xu, J., Campbell, D. A., and Wu, H. (2018). Global warming interacts with ocean acidification to alter PSII function and protection in the diatom Thalassiosira weissflogii. Environ. Exp. Bot. 147, 95–103. doi: 10.1016/j.envexpbot.2017.11.014
Garcia, C. A., Baer, S. E., Garcia, N. S., Rauschenberg, S., Twining, B. S., Lomas, M. W., et al. (2018). Nutrient supply controls particulate elemental concentrations and ratios in the low latitude eastern Indian Ocean. Nat. Commun. 9:4868. doi: 10.1038/s41467-018-06892-w
Geider, R. J., and La Roche, J. (2002). Redfield revisited: variability of C:N:P in marine microalgae and its biochemical basis. Eur. J. Phycol. 37, 1–17. doi: 10.1017/s0967026201003456
Gerhard, M., Koussoroplis, A. M., Hillebrand, H., and Striebel, M. (2019). Phytoplankton community responses to temperature fluctuations under different nutrient concentrations and stoichiometry. Ecology 100:e02834. doi: 10.1002/ecy.2834
Gettings, R. M., Townsend, D. W., Thomas, M. A., and Karp-Boss, L. (2014). Dynamics of late spring and summer phytoplankton communities on Georges Bank, with emphasis on diatoms, Alexandrium spp., and other dinoflagellates. Deep Sea Res. II Top. Stud. Oceanogr. 103, 120–138. doi: 10.1016/j.dsr2.2013.05.012
Glibert, P. M. (2020). Harmful algae at the complex nexus of eutrophication and climate change. Harmful Algae 91:101583. doi: 10.1016/j.hal.2019.03.001
Glibert, P. M., Fullerton, D., Burkholder, J. M., Cornwell, J. C., and Kana, T. M. (2011). Ecological stoichiometry, biogeochemical cycling, invasive species, and aquatic food webs: San Francisco estuary and comparative systems. Rev. Fish. Sci. 19, 358–417. doi: 10.1080/10641262.2011.611916
Grzebyk, D., and Berland, B. (1996). Influences of temperature, salinity and irradiance on growth of Prorocentrum minimum (Dinophyceae) from the Mediterranean Sea. J. Plankton Res. 18, 1837–1849. doi: 10.1093/plankt/18.10.1837
Guo, S., Feng, Y., Wang, L., Dai, M., Liu, Z., Bai, Y., et al. (2014a). Seasonal variation in the phytoplankton community of a continental-shelf sea: the East China Sea. Mar. Ecol. Prog. Ser. 516, 103–126. doi: 10.3354/meps10952
Guo, S., Li, Y., Zhang, C., Zhai, W., Huang, T., Wang, L., et al. (2014b). Phytoplankton community in the Bohai Sea and its relationship with environmental factors. Mar. Sci. Bull. 33, 95–105. (In Chinese with English abtract)
Guschina, I. A., and Harwood, J. L. (2009). “Algal lipids and effect of the environment on their biochemistry,” in Lipids in Aquatic Ecosystems, eds M. T. Arts, M. T. Brett, and M. J. Kainz (Heidelberg: Springer), 1–24.
Hansen, H. P., and Koroleff, F. (1999). “Determination of nutrients,” in Methods of Seawater Analysis, 3rd Edn, eds K. Grasshoff, K. Kremling, and M. Ehrhardt (Weinheim: WILEY-VCH), 159–228.
Harrison, P., and Berges, J. A. (2005). “Marine culture media,” in Algal Culturing Techniques, ed. R. A. Andersen (San Diego, CA: Academic Press), 578.
Heil, C. A., Glibert, P. M., and Fan, C. L. (2005). Prorocentrum minimum (Pavillard) Schiller – a review of a harmful algal bloom species of growing worldwide importance. Harmful Algae 4, 449–470. doi: 10.1016/j.hal.2004.08.003
Heisler, J., Glibert, P. M., Burkholder, J. M., Anderson, D. M., Cochlan, W., Dennison, W. C., et al. (2008). Eutrophication and harmful algal blooms: a scientific consensus. Harmful Algae 8, 3–13. doi: 10.1016/j.hal.2008.08.006
Hernandez, M. T., Mills, R. A., and Pancost, R. D. (2008). Algal biomarkers in surface waters around the Crozet plateau. Org. Geochem. 39, 1051–1057. doi: 10.1016/j.orggeochem.2008.04.015
Hinder, S. L., Hays, G. C., Edwards, M., Roberts, E. C., Walne, A. W., and Gravenor, M. B. (2012). Changes in marine dinoflagellate and diatom abundance under climate change. Nat. Clim. Change 2, 271–275. doi: 10.1038/nclimate1388
Hixson, S. M., and Arts, M. T. (2016). Climate warming is predicted to reduce omega-3, long-chain, polyunsaturated fatty acid production in phytoplankton. Glob. Change Biol. 22, 2744–2755. doi: 10.1111/gcb.13295
Hodgkiss, I. J., and Ho, K. C. (1997). Are changes in N:P ratios in coastal waters the key to increased red tide blooms? Hydrobiologia 352, 141–147. doi: 10.1023/a:1003046516964
Huang, W., Zhu, X., Zeng, J., He, Q., Xu, X., Liu, J., et al. (2012). Responses in growth and succession of the phytoplankton community to different N/P ratios near Dongtou Island in the East China Sea. J. Exp. Mar. Biol. Ecol. 434, 102–109. doi: 10.1016/j.jembe.2012.08.008
Ismar, S. M. H., Hansen, T., and Sommer, U. (2008). Effect of food concentration and type of diet on Acartia survival and naupliar development. Mar. Biol. 154, 335–343. doi: 10.1007/s00227-008-0928-9
Jiang, X., Han, Q., Gao, X., and Gao, G. (2016). Conditions optimising on the yield of biomass, total lipid, and valuable fatty acids in two strains of Skeletonema menzelii. Food Chem. 194, 723–732. doi: 10.1016/j.foodchem.2015.08.073
Jiang, Z., Chen, J., Gao, Y., Zhai, H., Jin, H., Zhou, F., et al. (2019). Regulation of spatial changes in phytoplankton community by water column stability and nutrients in the southern Yellow Sea. J. Geophys. Res. Biogeosci. 124, 2610–2627. doi: 10.1029/2018jg004785
Jiang, Z., Chen, J., Zhou, F., Shou, L., Chen, Q., Tao, B., et al. (2015). Controlling factors of summer phytoplankton community in the Changjiang (Yangtze River) Estuary and adjacent East China Sea shelf. Cont. Shelf Res. 101, 71–84. doi: 10.1016/j.csr.2015.04.009
Jo, N., La, H. S., Kim, J.-H., Kim, K., Kim, B. K., Kim, M. J., et al. (2021). Different biochemical compositions of particulate organic matter driven by major phytoplankton communities in the northwestern Ross Sea. Front. Microbiol. 12:623600. doi: 10.3389/fmicb.2021.623600
Jónasdóttir, S. H. (2019). Fatty acid profiles and production in marine phytoplankton. Mar. Drugs 17:151. doi: 10.3390/md17030151
Jónasdóttir, S. H., Visser, A. W., and Jespersen, C. (2009). Assessing the role of food quality in the production and hatching of Temora longicornis eggs. Mar. Ecol. Prog. Ser. 382, 139–150. doi: 10.3354/meps07985
Kainz, M., Arts, M. T., and Mazumder, A. (2004). Essential fatty acids in the planktonic food web and their ecological role for higher trophic levels. Limnol. Oceanogr. 49, 1784–1793. doi: 10.4319/lo.2004.49.5.1784
Klais, R., Norros, V., Lehtinen, S., Tamminen, T., and Olli, K. (2017). Community assembly and drivers of phytoplankton functional structure. Funct. Ecol. 31, 760–767. doi: 10.1111/1365-2435.12784
Klausmeier, C. A., Litchman, E., Daufresne, T., and Levin, S. A. (2004). Optimal nitrogen-to-phosphorus stoichiometry of phytoplankton. Nature 429, 171–174. doi: 10.1038/nature02454
Kohlbach, D., Hop, H., Wold, A., Schmidt, K., Smik, L., Belt, S. T., et al. (2021). Multiple trophic markers trace dietary carbon sources in Barents Sea zooplankton during late summer. Front. Mar. Sci. 7:610248. doi: 10.3389/fmars.2020.610248
Lassen, M. K., Nielsen, K. D., Richardson, K., Garde, K., and Schluter, L. (2010). The effects of temperature increases on a temperate phytoplankton community – a mesocosm climate change scenario. J. Exp. Mar. Biol. Ecol. 383, 79–88. doi: 10.1016/j.jembe.2009.10.014
Lavaud, J., Rousseau, B., and Etienne, A. L. (2004). General features of photoprotection by energy dissipation in planktonic diatoms (Bacillariophyceae). J. Phycol. 40, 130–137. doi: 10.1046/j.1529-8817.2004.03026.x
Lee, J. A., Garcia, C. A., Larkin, A. A., Carter, B. R., and Martiny, A. C. (2021). Linking a latitudinal gradient in ocean hydrography and elemental stoichiometry in the eastern Pacific Ocean. Glob. Biogeochem. Cycles 35:e2020GB006622. doi: 10.1029/2020gb006622
Leterme, S. C., Edwards, M., Seuront, L., Attrill, M. J., Reid, P. C., and John, A. W. G. (2005). Decadal basin-scale changes in diatoms, dinoflagellates, and phytoplankton color across the North Atlantic. Limnol. Oceanogr. 50, 1244–1253. doi: 10.4319/lo.2005.50.4.1244
Levitan, O., Dinamarca, J., Zelzion, E., Lun, D. S., Guerra, L. T., Kim, M. K., et al. (2015). Remodeling of intermediate metabolism in the diatom Phaeodactylum tricornutum under nitrogen stress. Proc. Natl. Acad. Sci. U.S.A. 112, 412–417. doi: 10.1073/pnas.1419818112
Lewandowska, A. M., Boyce, D. G., Hofmann, M., Matthiessen, B., Sommer, U., and Worm, B. (2014). Effects of sea surface warming on marine plankton. Ecol. Lett. 17, 614–623. doi: 10.1111/ele.12265
Liefer, J. D., Garg, A., Fyfe, M. H., Irwin, A. J., Benner, I., Brown, C. M., et al. (2019). The macromolecular basis of phytoplankton C:N:P under nitrogen starvation. Front. Microbiol. 10:763. doi: 10.3389/fmicb.2019.00763
Lin, S., Litaker, R. W., and Sunda, W. G. (2016). Phosphorus physiological ecology and molecular mechanisms in marine phytoplankton. J. Phycol. 52, 10–36. doi: 10.1111/jpy.12365
Liu, X., Xiao, W., Landry, M. R., Chiang, K.-P., Wang, L., and Huang, B. (2016). Responses of phytoplankton communities to environmental variability in the East China Sea. Ecosystems 19, 832–849. doi: 10.1007/s10021-016-9970-5
Loladze, I., and Elser, J. J. (2011). The origins of the Redfield nitrogen-to-phosphorus ratio are in a homoeostatic protein-to-rRNA ratio. Ecol. Lett. 14, 244–250. doi: 10.1111/j.1461-0248.2010.01577.x
Lotze, H. K., and Milewski, I. (2004). Two centuries of multiple human impacts and successive changes in a North Atlantic food web. Ecol. Appl. 14, 1428–1447. doi: 10.1890/03-5027
Malone, T. C. (1992). “Effects of water column processes on dissolved oxygen, nutrients, phytoplankton and zooplankton,” in Oxygen Dynamics in the Chesapeake Bay, eds D. A. Smith, M. Leffler, and G. Mackiernan (College Park, MD: Maryland Sea Grant College), 61–112.
Malzahn, A. M., and Boersma, M. (2012). Effects of poor food quality on copepod growth are dose dependent and non-reversible. Oikos 121, 1408–1416. doi: 10.1111/j.1600-0706.2011.20186.x
Malzahn, A. M., Hantzsche, F., Schoo, K. L., Boersma, M., and Aberle, N. (2010). Differential effects of nutrient-limited primary production on primary, secondary or tertiary consumers. Oecologia 162, 35–48. doi: 10.1007/s00442-009-1458-y
Margalef, R. (1978). Life-forms of phytoplankton as survival alternatives in an unstable environment. Oceanol. Acta 1, 493–509.
Martin, P., Dyhrman, S. T., Lomas, M. W., Poulton, N. J., and Van Mooy, B. A. S. (2014). Accumulation and enhanced cycling of polyphosphate by Sargasso Sea plankton in response to low phosphorus. Proc. Natl. Acad. Sci. U.S.A. 111, 8089–8094. doi: 10.1073/pnas.1321719111
Martin-Creuzburg, D., and von Elert, E. (2009). “Ecological significance of sterols in aquatic food webs,” in Lipids in Aquatic Ecosystems, eds M. T. Arts, M. T. Brett, and M. Kainz (Heidelberg: Springer), 43–64.
Martiny, A. C., Pham, C. T. A., Primeau, F. W., Vrugt, J. A., Moore, J. K., Levin, S. A., et al. (2013). Strong latitudinal patterns in the elemental ratios of marine plankton and organic matter. Nat. Geosci. 6, 279–283. doi: 10.1038/ngeo1757
Martiny, A. C., Talarmin, A., Mouginot, C., Lee, J. A., Huang, J. S., Gellene, A. G., et al. (2016). Biogeochemical interactions control a temporal succession in the elemental composition of marine communities. Limnol. Oceanogr. 61, 531–542. doi: 10.1002/lno.10233
Mills, M. M., and Arrigo, K. R. (2010). Magnitude of oceanic nitrogen fixation influenced by the nutrient uptake ratio of phytoplankton. Nat. Geosci. 3, 412–416. doi: 10.1038/ngeo856
Montero, P., Perez-Santos, I., Daneri, G., Gutierrez, M. H., Igor, G., Seguel, R., et al. (2017). A winter dinoflagellate bloom drives high rates of primary production in a Patagonian fjord ecosystem. Estuar. Coast. Shelf Sci. 199, 105–116. doi: 10.1016/j.ecss.2017.09.027
Moore, C. M., Mills, M. M., Arrigo, K. R., Berman-Frank, I., Bopp, L., Boyd, P. W., et al. (2013). Processes and patterns of oceanic nutrient limitation. Nat. Geosci. 6, 701–710. doi: 10.1038/ngeo1765
Morse, R. E., Mulholland, M. R., Hunley, W. S., Fentress, S., Wiggins, M., and Blanco-Garcia, J. L. (2013). Controls on the initiation and development of blooms of the dinoflagellate Cochlodinium polykrikoides Margalef in lower Chesapeake Bay and its tributaries. Harmful Algae 28, 71–82. doi: 10.1016/j.hal.2013.05.013
Müller-Navarra, D. C., Brett, M. T., Liston, A. M., and Goldman, C. R. (2000). A highly unsaturated fatty acid predicts carbon transfer between primary producers and consumers. Nature 403, 74–77. doi: 10.1038/47469
Mutshinda, C. M., Finkel, Z. V., Widdicombe, C. E., and Irwin, A. J. (2016). Ecological equivalence of species within phytoplankton functional groups. Funct. Ecol. 30, 1714–1722. doi: 10.1111/1365-2435.12641
Provasoli, L. (1963). “Growing marine seaweeds,” in Proceedings of the 4th International. Seaweed Symposium, eds A. D. De Virville and J. Feldmann (Oxford: Pergamon Press), 9–17.
Quigg, A., Finkel, Z. V., Irwin, A. J., Rosenthal, Y., Ho, T.-Y., Reinfelder, J. R., et al. (2003). The evolutionary inheritance of elemental stoichiometry in marine phytoplankton. Nature 425, 291–294. doi: 10.1038/nature01953
R Development Core Team (2010). R: A Language and Environment for Statistical Computing. Vienna: R Foundation for Statistical Computing.
Ratmaya, W., Soudant, D., Salmon-Monviola, J., Plus, M., Cochennec-Laureau, N., Goubert, E., et al. (2019). Reduced phosphorus loads from the Loire and Vilaine rivers were accompanied by increasing eutrophication in the Vilaine Bay (south Brittany, France). Biogeosciences 16, 1361–1380. doi: 10.5194/bg-16-1361-2019
Reinfelder, J. R. (2012). Carbon dioxide regulation of nitrogen and phosphorus in four species of marine phytoplankton. Mar. Ecol. Prog. Ser. 466, 57–67. doi: 10.3354/meps09905
Reuss, N. R., and Poulsen, L. P. (2002). Evaluation of fatty acids as biomarkers for a natural plankton community. A field study of a spring bloom and a post-bloom period off West Greenland. Mar. Biol. 141, 423–434. doi: 10.1007/s00227-002-0841-6
Rousseaux, C. S., and Gregg, W. W. (2015). Recent decadal trends in global phytoplankton composition. Glob. Biogeochem. Cycles 29, 1674–1688. doi: 10.1002/2015gb005139
Ruess, L., and Müller-Navarra, D. C. (2019). Essential biomolecules in food webs. Front. Ecol. Evol. 7:269. doi: 10.3389/fevo.2019.00269
Schubert, C. J., Villanueva, J., Calvert, S. E., Cowie, G. L., von Rad, U., Schulz, H., et al. (1998). Stable phytoplankton community structure in the Arabian Sea over the past 200,000 years. Nature 394, 563–566. doi: 10.1038/29047
Sharoni, S., and Halevy, I. (2020). Nutrient ratios in marine particulate organic matter are predicted by the population structure of well-adapted phytoplankton. Sci. Adv. 6:eaaw9371. doi: 10.1126/sciadv.aaw9371
Sharp, J. (1974). Improved analysis for particulate organic carbon and nitrogen from seawater. Limnol. Oceanogr. 19, 984–989. doi: 10.4319/lo.1974.19.6.0984
Shemi, A., Schatz, D., Fredricks, H. F., Van Mooy, B. A. S., Porat, Z., and Vardi, A. (2016). Phosphorus starvation induces membrane remodeling and recycling in Emiliania huxleyi. New Phytol. 211, 886–898. doi: 10.1111/nph.13940
Siemering, B., Bresnan, E., Painter, S. C., Daniels, C. J., Inall, M., and Davidson, K. (2016). Phytoplankton distribution in relation to environmental drivers on the North West European Shelf sea. PLoS One 11:e0164482. doi: 10.1371/journal.pone.0164482
Smayda, T. J. (2002). Adaptive ecology, growth strategies and the global bloom expansion of dinoflagellates. J. Oceanogr. 58, 281–294. doi: 10.1023/a:1015861725470
Smayda, T. J., and Reynolds, C. S. (2003). Strategies of marine dinoflagellate survival and some rules of assembly. J. Sea Res. 49, 95–106. doi: 10.1016/s1385-1101(02)00219-8
Smith, S. R., Dupont, C. L., McCarthy, J. K., Broddrick, J. T., Obornik, M., Horak, A., et al. (2019). Evolution and regulation of nitrogen flux through compartmentalized metabolic networks in a marine diatom. Nat. Commun. 10:4552. doi: 10.1038/s41467-019-12407-y
Sommer, U. (1989). “The role of competition for resources in phytoplankton succession,” in Plankton Ecology, ed. U. Sommer (Berlin: Springer), 57–106.
Sommer, U., Stibor, H., Katechakis, A., Sommer, F., and Hansen, T. (2002). Pelagic food web configurations at different levels of nutrient richness and their implications for the ratio fish production: primary production. Hydrobiologia 484, 11–20. doi: 10.1023/a:1021340601986
Spilling, K., Olli, K., Lehtoranta, J., Kremp, A., Tedesco, L., Tamelander, T., et al. (2018). Shifting diatom-dinoflagellate dominance during spring bloom in the Baltic Sea and its potential effects on biogeochemical cycling. Front. Mar. Sci. 5:327. doi: 10.3389/fmars.2018.00327
Sterner, R. W., and Elser, J. J. (2002). Ecological Stoichiometry: The Biology of Elements from Molecules to the Biosphere. Princeton, NJ: Princeton University Press.
Sunagawa, S., Coelho, L. P., Chaffron, S., Kultima, J. R., Labadie, K., Salazar, G., et al. (2015). Structure and function of the global ocean microbiome. Science 348:1261359. doi: 10.1126/science.1261359
Tameishi, M., Yamasaki, Y., Nagasoe, S., Shimasaki, Y., Oshima, Y., and Honjo, T. (2009). Allelopathic effects of the dinophyte Prorocentrum minimum on the growth of the bacillariophyte Skeletonema costatum. Harmful Algae 8, 421–429. doi: 10.1016/j.hal.2008.09.002
Tanioka, T., and Matsumoto, K. (2020). A meta-analysis on environmental drivers of marine phytoplankton C:N:P. Biogeosciences 17, 2939–2954. doi: 10.5194/bg-17-2939-2020
Thrane, J.-E., Hessen, D. O., and Andersen, T. (2017). Plasticity in algal stoichiometry: experimental evidence of a temperature-induced shift in optimal supply N:P ratio. Limnol. Oceanogr. 62, 1346–1354. doi: 10.1002/lno.10500
Tilman, D. (1982). Resource Competition and Community Structure. Princeton, NJ: Princeton University Press.
Toseland, A., Daines, S. J., Clark, J. R., Kirkham, A., Strauss, J., Uhlig, C., et al. (2013). The impact of temperature on marine phytoplankton resource allocation and metabolism. Nat. Clim. Change 3, 979–984. doi: 10.1038/nclimate1989
Trommer, G., Lorenz, P., Lentz, A., Fink, P., and Stibor, H. (2019). Nitrogen enrichment leads to changing fatty acid composition of phytoplankton and negatively affects zooplankton in a natural lake community. Sci. Rep. 9:16805. doi: 10.1038/s41598-019-53250-x
Tyrrell, T. (1999). The relative influences of nitrogen and phosphorus on oceanic primary production. Nature 400, 525–531. doi: 10.1038/22941
Volkman, J. K. (2003). Sterols in microorganisms. Appl. Mirobiol. Biotechnol. 60, 495–506. doi: 10.1007/s00253-002-1172-8
Wang, Z., Li, R., Zhu, M., Chen, B., and Hao, Y. (2006). Study on population growth processes and interspecific competition of Prorocentrum donghaiense and Skeletonema costatum in semi-continuous dilution experiments. Adv. Mar. Sci. 24, 495–503. (In Chinese with English abstract)
Wang, Z., Xiao, X., Yuan, Z., Wang, F., Xing, L., Gong, X., et al. (2019). Air-sea interactive forcing on phytoplankton productivity and community structure changes in the East China Sea during the Holocene. Glob. Planet. Change 179, 80–91. doi: 10.1016/j.gloplacha.2019.05.008
Weber, T. S., and Deutsch, C. (2010). Ocean nutrient ratios governed by plankton biogeography. Nature 467, 550–554. doi: 10.1038/nature09403
White, D. A., Widdicombe, C. E., Somerfield, P. J., Airs, R. L., Tarran, G. A., Maud, J. L., et al. (2015). The combined effects of seasonal community succession and adaptive algal physiology on lipid profiles of coastal phytoplankton in the Western English Channel. Mar. Chem. 177, 638–652. doi: 10.1016/j.marchem.2015.10.005
William, K. W. L., and Morris, I. (1982). Temperature adaptation in Phaeodactylum tricornutum Bohlin: photosynthetic rate compensation and capacity. J. Exp. Mar. Biol. Ecol. 58, 135–150. doi: 10.1016/0022-0981(82)90125-3
Wood, S. N. (2017). Generalized Additive Models: An Introduction with R, 2nd Edn. Boca Raton, FL: Chapman & Hall/CRC.
Wu, P., Bi, R., Duan, S., Jin, H., Chen, J., Hao, Q., et al. (2016). Spatiotemporal variations of phytoplankton in the East China Sea and the Yellow Sea revealed by lipid biomarkers. J. Geophys. Res. Biogeosci. 121, 109–125. doi: 10.1002/2015jg003167
Xiao, W., Liu, X., and Huang, B. (2013). Phytoplankton community structure and its environmental controlling in the nearshore surface water of the East China Sea in spring. J. Mar. Sci. 31, 76–82. (In Chinese with English abstract)
Xiao, W., Liu, X., Irwin, A. J., Laws, E. A., Wang, L., Chen, B., et al. (2018). Warming and eutrophication combine to restructure diatoms and dinoflagellates. Water Res. 128, 206–216. doi: 10.1016/j.watres.2017.10.051
Xie, Y., Tilstone, G. H., Widdicombe, C., Woodward, E. M. S., Harris, C., and Barnes, M. K. (2015). Effect of increases in temperature and nutrients on phytoplankton community structure and photosynthesis in the western English Channel. Mar. Ecol. Prog. Ser. 519, 61–73. doi: 10.3354/meps11101
Xing, L., Zhao, M., Zhang, T., Yu, M., Duan, S., Zhang, R., et al. (2016). Ecosystem responses to anthropogenic and natural forcing over the last 100 years in the coastal areas of the East China Sea. Holocene 26, 669–677. doi: 10.1177/0959683615618248
Yuan, Z., Liu, D., Masqué, P., Zhao, M., Song, X., and Keesing, J. K. (2020). Phytoplankton responses to climate-induced warming and interdecadal oscillation in north-western Australia. Paleoceanogr. Paleoclimatol. 35:e2019A003712. doi: 10.1029/2019pa003712
Yvon-Durocher, G., Dossena, M., Trimmer, M., Woodward, G., and Allen, A. P. (2015). Temperature and the biogeography of algal stoichiometry. Glob. Ecol. Biogeogr. 24, 562–570. doi: 10.1111/geb.12280
Zhang, F. (2009). Historical Comparison on Evolution of Phytoplankton Community and Its Relations with Environmental Factors in Yangtze Estuary and Its Adjacent Sea. Master’s thesis. Shanghai: East China Normal University.
Zhao, M. X., Mercer, J. L., Eglinton, G., Higginson, M. J., and Huang, C.-Y. (2006). Comparative molecular biomarker assessment of phytoplankton paleoproductivity for the last 160 kyr off Cap Blanc, NW Africa. Org. Geochem. 37, 72–97.
Zhuang, Y., Zhang, H., Hannick, L., and Lin, S. (2015). Metatranscriptome profiling reveals versatile N-nutrient utilization, CO2 limitation, oxidative stress, and active toxin production in an Alexandrium fundyense bloom. Harmful Algae 42, 60–70. doi: 10.1016/j.hal.2014.12.006
Keywords: Phaeodactylum tricornutum, Prorocentrum minimum, elemental stoichiometry, fatty acids, sterols, temperature, nutrients
Citation: Bi R, Cao Z, Ismar-Rebitz SMH, Sommer U, Zhang H, Ding Y and Zhao M (2021) Responses of Marine Diatom-Dinoflagellate Competition to Multiple Environmental Drivers: Abundance, Elemental, and Biochemical Aspects. Front. Microbiol. 12:731786. doi: 10.3389/fmicb.2021.731786
Received: 28 June 2021; Accepted: 09 August 2021;
Published: 30 August 2021.
Edited by:
Yuanyuan Feng, Shanghai Jiao Tong University, ChinaCopyright © 2021 Bi, Cao, Ismar-Rebitz, Sommer, Zhang, Ding and Zhao. This is an open-access article distributed under the terms of the Creative Commons Attribution License (CC BY). The use, distribution or reproduction in other forums is permitted, provided the original author(s) and the copyright owner(s) are credited and that the original publication in this journal is cited, in accordance with accepted academic practice. No use, distribution or reproduction is permitted which does not comply with these terms.
*Correspondence: Rong Bi, rongbi@ouc.edu.cn; Meixun Zhao, maxzhao@ouc.edu.cn; maxzhao04@yahoo.com